
Want to create or adapt books like this? Learn more about how Pressbooks supports open publishing practices.

21 Mendel’s Experiments
By the end of this section, you will be able to:
- Explain the scientific reasons for the success of Mendel’s experimental work
- Describe the expected outcomes of monohybrid crosses involving dominant and recessive alleles
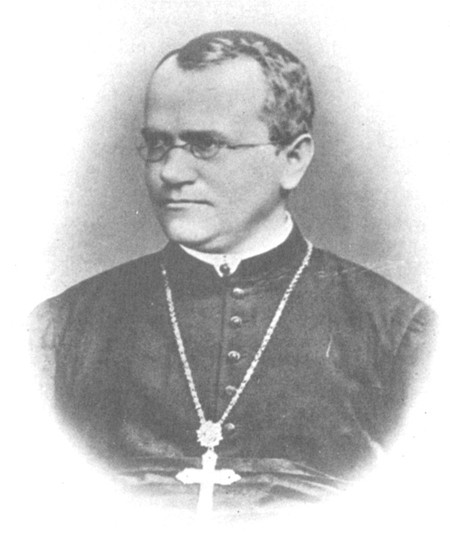
Johann Gregor Mendel (1822–1884) (Figure 1) was a lifelong learner, teacher, scientist, and man of faith. As a young adult, he joined the Augustinian Abbey of St. Thomas in Brno in what is now the Czech Republic. Supported by the monastery, he taught physics, botany, and natural science courses at the secondary and university levels. In 1856, he began a decade-long research pursuit involving inheritance patterns in honeybees and plants, ultimately settling on pea plants as his primary model system (a system with convenient characteristics that is used to study a specific biological phenomenon to gain understanding to be applied to other systems). In 1865, Mendel presented the results of his experiments with nearly 30,000 pea plants to the local natural history society. He demonstrated that traits are transmitted faithfully from parents to offspring in specific patterns. In 1866, he published his work, Experiments in Plant Hybridization, 1 in the proceedings of the Natural History Society of Brünn.
Mendel’s work went virtually unnoticed by the scientific community, which incorrectly believed that the process of inheritance involved a blending of parental traits that produced an intermediate physical appearance in offspring. This hypothetical process appeared to be correct because of what we know now as continuous variation. Continuous variation is the range of small differences we see among individuals in a characteristic like human height. It does appear that offspring are a “blend” of their parents’ traits when we look at characteristics that exhibit continuous variation. Mendel worked instead with traits that show discontinuous variation . Discontinuous variation is the variation seen among individuals when each individual shows one of two—or a very few—easily distinguishable traits, such as violet or white flowers. Mendel’s choice of these kinds of traits allowed him to see experimentally that the traits were not blended in the offspring as would have been expected at the time, but that they were inherited as distinct traits. In 1868, Mendel became abbot of the monastery and exchanged his scientific pursuits for his pastoral duties. He was not recognized for his extraordinary scientific contributions during his lifetime; in fact, it was not until 1900 that his work was rediscovered, reproduced, and revitalized by scientists on the brink of discovering the chromosomal basis of heredity.
Mendel’s Crosses
Mendel’s seminal work was accomplished using the garden pea, Pisum sativum , to study inheritance. This species naturally self-fertilizes, meaning that pollen encounters ova within the same flower. The flower petals remain sealed tightly until pollination is completed to prevent the pollination of other plants. The result is highly inbred, or “true-breeding,” pea plants. These are plants that always produce offspring that look like the parent. By experimenting with true-breeding pea plants, Mendel avoided the appearance of unexpected traits in offspring that might occur if the plants were not true-breeding. The garden pea also grows to maturity within one season, meaning that several generations could be evaluated over a relatively short time. Finally, large quantities of garden peas could be cultivated simultaneously, allowing Mendel to conclude that his results did not come about simply by chance.
Mendel performed hybridizations , which involve mating two true-breeding individuals that have different traits. In the pea, which is naturally self-pollinating, this is done by manually transferring pollen from the anther of a mature pea plant of one variety to the stigma of a separate mature pea plant of the second variety.
Plants used in first-generation crosses were called P , or parental generation, plants (Figure 2). Mendel collected the seeds produced by the P plants that resulted from each cross and grew them the following season. These offspring were called the F 1 , or the first filial (filial = daughter or son), generation. Once Mendel examined the characteristics in the F 1 generation of plants, he allowed them to self-fertilize naturally. He then collected and grew the seeds from the F 1 plants to produce the F 2 , or second filial, generation. Mendel’s experiments extended beyond the F 2 generation to the F 3 generation, F 4 generation, and so on, but it was the ratio of characteristics in the P, F 1 , and F 2 generations that were the most intriguing and became the basis of Mendel’s postulates.
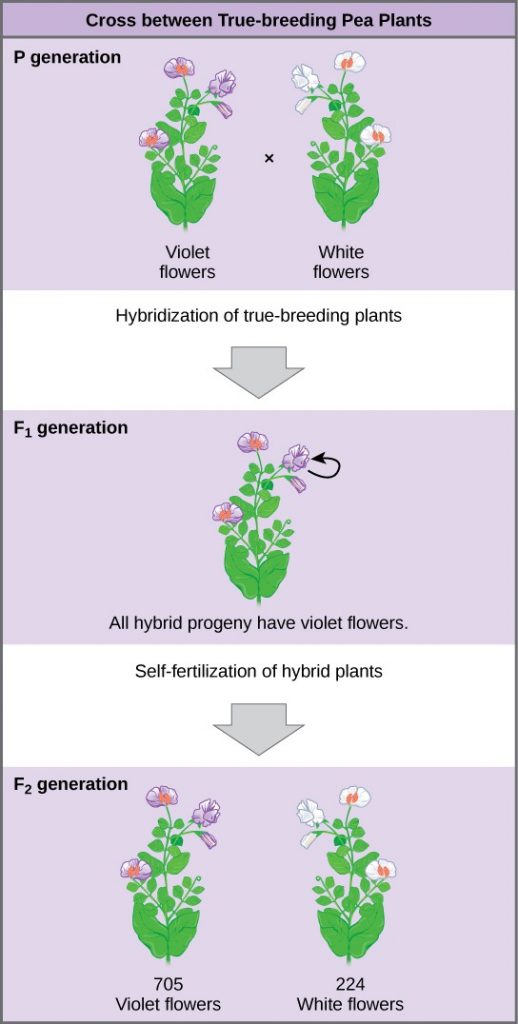
Garden Pea Characteristics Revealed the Basics of Heredity
In his 1865 publication, Mendel reported the results of his crosses involving seven different characteristics, each with two contrasting traits. A trait is defined as a variation in the physical appearance of a heritable characteristic. The characteristics included plant height, seed texture, seed color, flower color, pea-pod size, pea-pod color, and flower position. For the characteristic of flower color, for example, the two contrasting traits were white versus violet. To fully examine each characteristic, Mendel generated large numbers of F 1 and F 2 plants and reported results from thousands of F 2 plants.
What results did Mendel find in his crosses for flower color? First, Mendel confirmed that he was using plants that bred true for white or violet flower color. Irrespective of the number of generations that Mendel examined, all self-crossed offspring of parents with white flowers had white flowers, and all self-crossed offspring of parents with violet flowers had violet flowers. In addition, Mendel confirmed that, other than flower color, the pea plants were physically identical. This was an important check to make sure that the two varieties of pea plants only differed with respect to one trait, flower color.
Once these validations were complete, Mendel applied the pollen from a plant with violet flowers to the stigma of a plant with white flowers. After gathering and sowing the seeds that resulted from this cross, Mendel found that 100 percent of the F 1 hybrid generation had violet flowers. Conventional wisdom at that time would have predicted the hybrid flowers to be pale violet or for hybrid plants to have equal numbers of white and violet flowers. In other words, the contrasting parental traits were expected to blend in the offspring. Instead, Mendel’s results demonstrated that the white flower trait had completely disappeared in the F 1 generation.
Importantly, Mendel did not stop his experimentation there. He allowed the F 1 plants to self-fertilize and found that 705 plants in the F 2 generation had violet flowers and 224 had white flowers. This was a ratio of 3.15 violet flowers to one white flower, or approximately 3:1. When Mendel transferred pollen from a plant with violet flowers to the stigma of a plant with white flowers and vice versa, he obtained approximately the same ratio irrespective of which parent—male or female—contributed which trait. This is called a reciprocal cross —a paired cross in which the respective traits of the male and female in one cross become the respective traits of the female and male in the other cross. For the other six characteristics that Mendel examined, the F 1 and F 2 generations behaved in the same way that they behaved for flower color. One of the two traits would disappear completely from the F 1 generation, only to reappear in the F 2 generation at a ratio of roughly 3:1 (Figure 3).
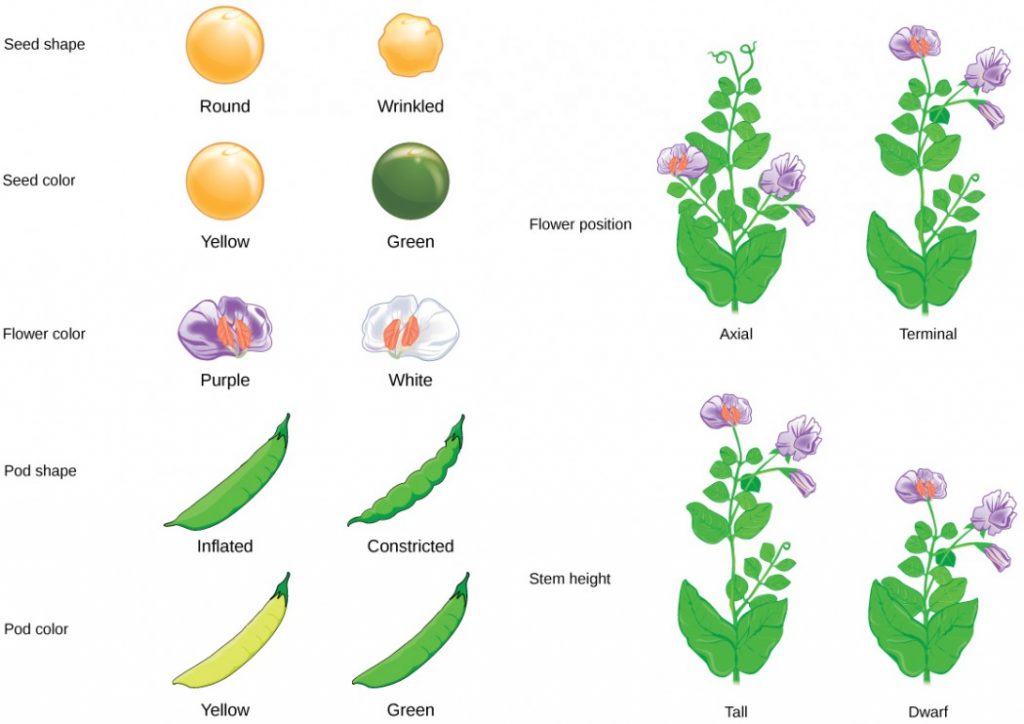
Upon compiling his results for many thousands of plants, Mendel concluded that the characteristics could be divided into expressed and latent traits. He called these dominant and recessive traits, respectively. Dominant traits are those that are inherited unchanged in a hybridization. Recessive traits become latent, or disappear in the offspring of a hybridization. The recessive trait does, however, reappear in the progeny of the hybrid offspring. An example of a dominant trait is the violet-colored flower trait. For this same characteristic (flower color), white-colored flowers are a recessive trait. The fact that the recessive trait reappeared in the F 2 generation meant that the traits remained separate (and were not blended) in the plants of the F 1 generation. Mendel proposed that this was because the plants possessed two copies of the trait for the flower-color characteristic, and that each parent transmitted one of their two copies to their offspring, where they came together. Moreover, the physical observation of a dominant trait could mean that the genetic composition of the organism included two dominant versions of the characteristic, or that it included one dominant and one recessive version. Conversely, the observation of a recessive trait meant that the organism lacked any dominant versions of this characteristic.
CONCEPTS IN ACTION
For an excellent review of Mendel’s experiments and to perform your own crosses and identify patterns of inheritance, visit the Mendel’s Peas web lab .
Also, check out the following video as review
- Johann Gregor Mendel, “Versuche über Pflanzenhybriden.” Verhandlungen des naturforschenden Vereines in Brünn , Bd. IV für das Jahr, 1865 Abhandlungen (1866):3–47. [for English translation, see http://www.mendelweb.org/Mendel.plain.html]
Introductory Biology: Evolutionary and Ecological Perspectives Copyright © by Various Authors - See Each Chapter Attribution is licensed under a Creative Commons Attribution 4.0 International License , except where otherwise noted.
Share This Book
NOTIFICATIONS
Mendel’s experiments.
- + Create new collection
Mendel is known as the father of genetics because of his ground-breaking work on inheritance in pea plants 150 years ago.
Gregor Johann Mendel was a monk and teacher with interests in astronomy and plant breeding. He was born in 1822, and at 21, he joined a monastery in Brünn (now in the Czech Republic). The monastery had a botanical garden and library and was a centre for science, religion and culture . In 1856, Mendel began a series of experiments at the monastery to find out how traits are passed from generation to generation. At the time, it was thought that parents’ traits were blended together in their progeny .
Studying traits in peas
Mendel studied inheritance in peas ( Pisum sativum ). He chose peas because they had been used for similar studies, are easy to grow and can be sown each year. Pea flowers contain both male and female parts, called stamen and stigma , and usually self-pollinate. Self-pollination happens before the flowers open, so progeny are produced from a single plant.
Peas can also be cross-pollinated by hand, simply by opening the flower buds to remove their pollen-producing stamen (and prevent self-pollination) and dusting pollen from one plant onto the stigma of another.
Traits in pea plants
Mendel followed the inheritance of 7 traits in pea plants, and each trait had 2 forms. He identified pure-breeding pea plants that consistently showed 1 form of a trait after generations of self-pollination.
Mendel then crossed these pure-breeding lines of plants and recorded the traits of the hybrid progeny. He found that all of the first-generation (F1) hybrids looked like 1 of the parent plants. For example, all the progeny of a purple and white flower cross were purple (not pink, as blending would have predicted). However, when he allowed the hybrid plants to self-pollinate, the hidden traits would reappear in the second-generation (F2) hybrid plants.
Dominant and recessive traits
Mendel described each of the trait variants as dominant or recessive Dominant traits, like purple flower colour, appeared in the F1 hybrids, whereas recessive traits, like white flower colour, did not.
Mendel did thousands of cross-breeding experiments. His key finding was that there were 3 times as many dominant as recessive traits in F2 pea plants (3:1 ratio).
Traits are inherited independently
Mendel also experimented to see what would happen if plants with 2 or more pure-bred traits were cross-bred. He found that each trait was inherited independently of the other and produced its own 3:1 ratio. This is the principle of independent assortment.
Find out more about Mendel’s principles of inheritance .
The next generations
Mendel didn’t stop there – he continued to allow the peas to self-pollinate over several years whilst meticulously recording the characteristics of the progeny. He may have grown as many as 30,000 pea plants over 7 years.
Mendel’s findings were ignored
In 1866, Mendel published the paper Experiments in plant hybridisation ( Versuche über plflanzenhybriden ). In it, he proposed that heredity is the result of each parent passing along 1 factor for every trait. If the factor is dominant , it will be expressed in the progeny. If the factor is recessive, it will not show up but will continue to be passed along to the next generation. Each factor works independently from the others, and they do not blend.
The science community ignored the paper, possibly because it was ahead of the ideas of heredity and variation accepted at the time. In the early 1900s, 3 plant biologists finally acknowledged Mendel’s work. Unfortunately, Mendel was not around to receive the recognition as he had died in 1884.
Useful links
Download a translated version of Mendel’s 1866 paper Experiments in plant hybridisation from Electronic Scholarly Publishing.
This apple cross-pollination video shows scientists at Plant & Food Research cross-pollinating apple plants.
See our newsletters here .
Would you like to take a short survey?
This survey will open in a new tab and you can fill it out after your visit to the site.
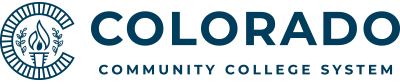
5.10 Mendel’s Experiments and Laws of Inheritance
Of peas and people.
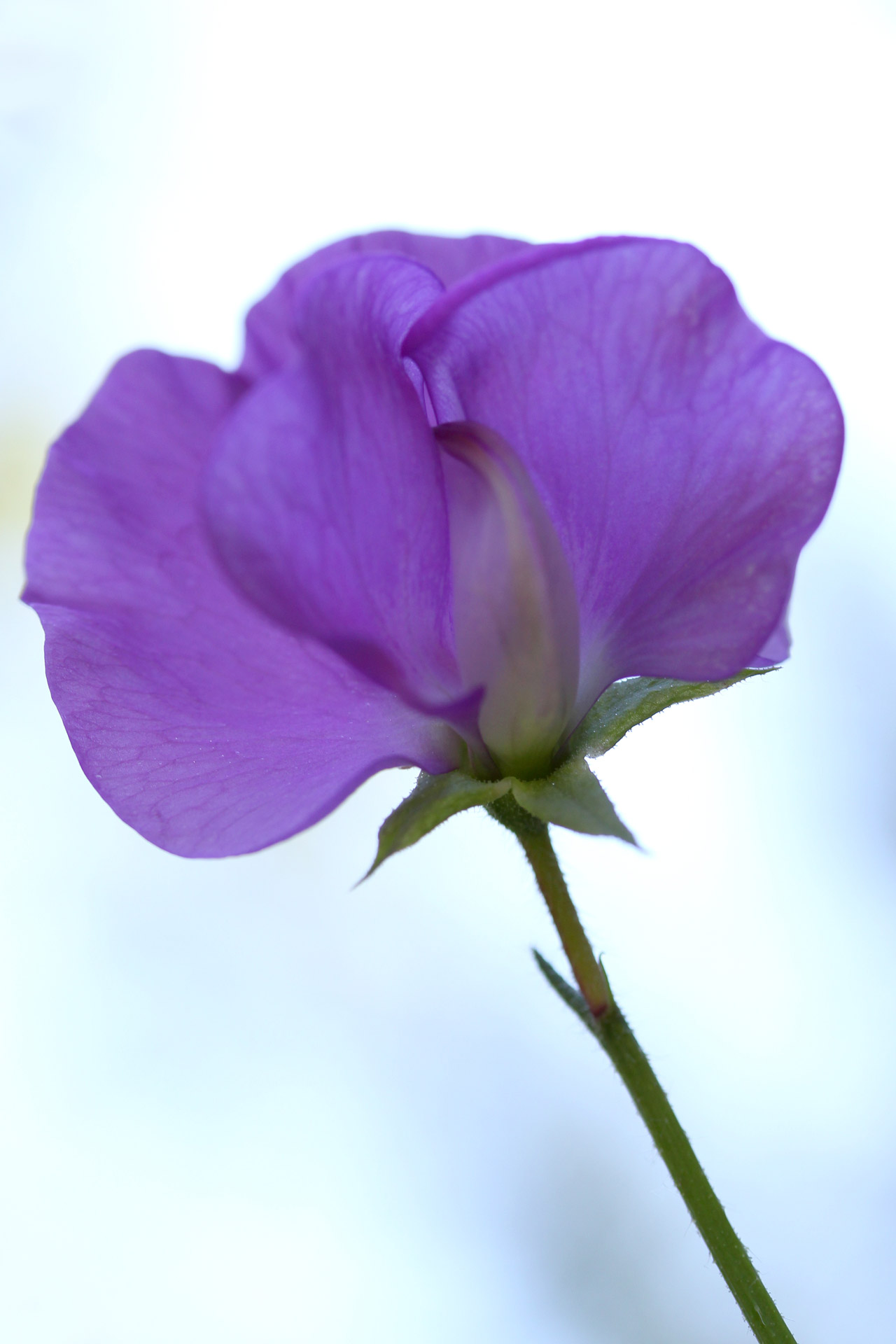
These purple-flowered plants are not just pretty to look at. Plants like these led to a huge leap forward in biology. They’re common garden peas, and they were studied in the mid-1800s by an Austrian monk named Gregor Mendel . Through careful experimentation, Mendel uncovered the secrets of heredity, or how parents pass characteristics to their offspring. You may not care much about heredity in pea plants, but you probably care about your own heredity. Mendel’s discoveries apply to people, as well as to peas — and to all other living things that reproduce sexually. In this concept, you will read about Mendel’s experiments and the secrets of heredity that he discovered.
Mendel and His Pea Plants
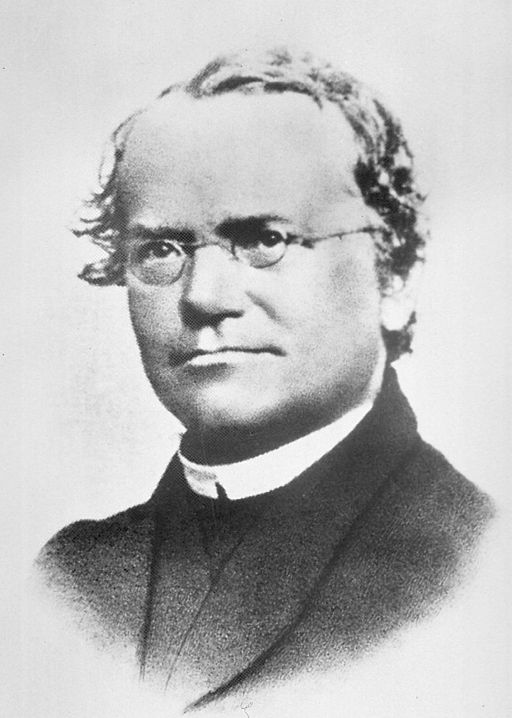
Gregor Mendel (Figure 5.10.2) was born in 1822. He grew up on his parents’ farm in Austria. He did well in school and became a friar (and later an abbot) at St. Thomas’ Abbey. Through sponsorship from the monastery, he went on to the University of Vienna, where he studied science and math. His professors encouraged him to learn science through experimentation, and to use math to make sense of his results. Mendel is best known for his experiments with pea plants (like the purple flower pictured in Figure 5.10.1).
Blending Theory of Inheritance
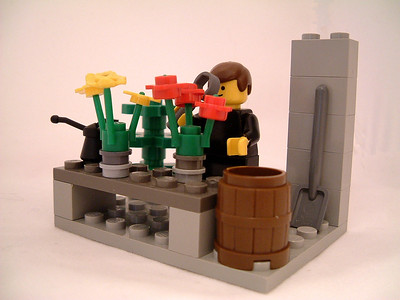
During Mendel’s time, the blending theory of inheritance was popular. According to this theory, offspring have a blend (or mix) of their parents’ characteristics. Mendel, however, noticed plants in his own garden that weren’t a blend of the parents. For example, a tall plant and a short plant had offspring that were either tall or short — not medium in height. Observations such as these led Mendel to question the blending theory. He wondered if there was a different underlying principle that could explain how characteristics are inherited. He decided to experiment with pea plants to find out. In fact, Mendel experimented with almost 30 thousand pea plants over the next several years!
Why Study Pea Plants?
Why did Mendel choose common, garden-variety pea plants for his experiments? Pea plants are a good choice because they are fast-growing and easy to raise. They also have several visible characteristics that can vary. These characteristics — some of which are illustrated in Figure 5.10.4 — include seed form and colour, flower colour, pod form and colour, placement of pods and flowers on stems, and stem length. Each of these characteristics has two common values. For example, seed form may be round or wrinkled, and flower colour may be white or purple (violet).
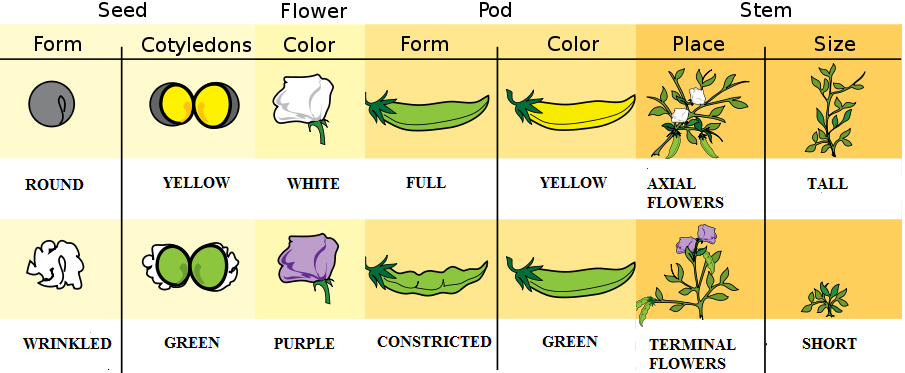
Controlling Pollination
To research how characteristics are passed from parents to offspring, Mendel needed to control pollination , which is the fertilization step in the sexual reproduction of plants. Pollen consists of tiny grains that are the male sex cells (or gametes) of plants. They are produced by a male flower part called the anther. Pollination occurs when pollen is transferred from the anther to the stigma of the same or another flower. The stigma is a female part of a flower, and it passes pollen grains to female gametes in the ovary.
Pea plants are naturally self-pollinating. In self-pollination , pollen grains from anthers on one plant are transferred to stigmas of flowers on the same plant. Mendel was interested in the offspring of two different parent plants, so he had to prevent self-pollination. He removed the anthers from the flowers of some of the plants in his experiments. Then he pollinated them by hand using a small paintbrush with pollen from other parent plants of his choice.
When pollen from one plant fertilizes another plant of the same species, it is called cross-pollination . The offspring that result from such a cross are called hybrids . When the term hybrid is used in this context, it refers to any offspring resulting from the breeding of two genetically distinct individuals.
Mendel’s First Set of Experiments
At first, Mendel experimented with just one characteristic at a time. He began with flower colour. As shown in Figure 5.10.5, Mendel cross-pollinated purple- and white-flowered parent plants. The parent plants in the experiments are referred to as the P (for parent) generation.
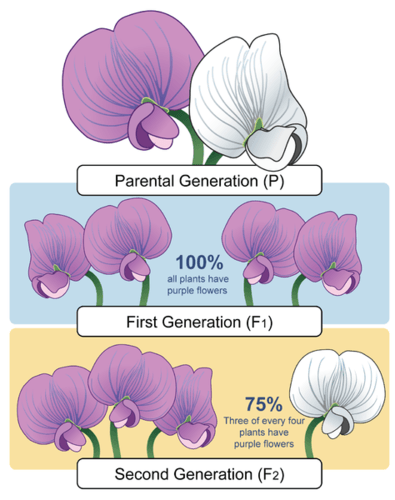
Figure 5.10.5 shows Mendel’s first experiment with pea plants. The F1 generation results from the cross-pollination of two parent (P) plants, and it contains all purple flowers. The F2 generation results from the self-pollination of F1 plants, and contains 75% purple flowers and 25% white flowers.
F1 and F2 Generations
The offspring of the P generation are called the F1 (for filial, or “offspring”) generation. As shown in Figure 5.10.5, all of the plants in the F1 generation had purple flowers — none of them had white flowers. Mendel wondered what had happened to the white-flower characteristic. He assumed that some type of inherited factor produces white flowers and some other inherited factor produces purple flowers. Did the white-flower factor just disappear in the F1 generation? If so, then the offspring of the F1 generation — called the F2 generation — should all have purple flowers like their parents.
To test this prediction, Mendel allowed the F1 generation plants to self-pollinate. He was surprised by the results. Some of the F2 generation plants had white flowers. He studied hundreds of F2 generation plants, and for every three purple-flowered plants, there was an average of one white-flowered plant.
Law of Segregation
Mendel did the same experiment for all seven characteristics. In each case, one value of the characteristic disappeared in the F1 plants, later showing up again in the F2 plants. In each case, 75 per cent of F2 plants had one value of the characteristic, while 25 per cent had the other value. Based on these observations, Mendel formulated his first law of inheritance. This law is called the law of segregation . It states that there are two factors controlling a given characteristic, one of which dominates the other, and these factors separate and go to different gametes when a parent reproduces.
Mendel’s Second Set of Experiments
Mendel wondered whether different characteristics are inherited together. For example, are purple flowers and tall stems always inherited together, or do these two characteristics show up in different combinations in offspring? To answer these questions, Mendel next investigated two characteristics at a time. For example, he crossed plants with yellow round seeds and plants with green wrinkled seeds. The results of this cross are shown in Figure 5.10.6.
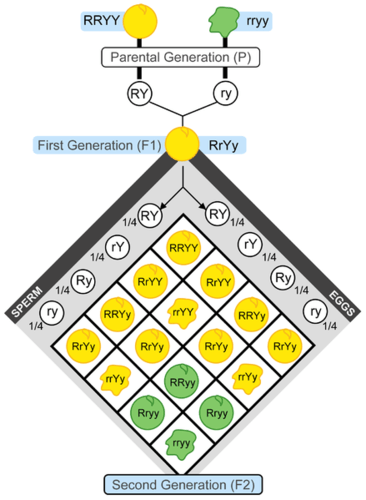
Figure 5.10.6 shows the outcome of a cross between plants that differ in seed colour (yellow or green) and seed form (shown here with a smooth round appearance or wrinkled appearance). The letters R, r, Y, and y represent genes for the characteristics Mendel was studying. Mendel didn’t know about genes, however, because genes would not be discovered until several decades later. This experiment demonstrates that, in the F2 generation, nine out of 16 were round yellow seeds, three out of 16 were wrinkled yellow seeds, three out of 16 were round green seeds, and one out of 16 was wrinkled green seeds.
In this set of experiments, Mendel observed that plants in the F1 generation were all alike. All of them had yellow round seeds like one of the two parents. When the F1 generation plants self-pollinated, however, their offspring — the F2 generation — showed all possible combinations of the two characteristics. Some had green round seeds, for example, and some had yellow wrinkled seeds. These combinations of characteristics were not present in the F1 or P generations.
Law of Independent Assortment
Mendel repeated this experiment with other combinations of characteristics, such as flower colour and stem length. Each time, the results were the same as those shown in Figure 5.10.6. The results of Mendel’s second set of experiments led to his second law. This is the law of independent assortment . It states that factors controlling different characteristics are inherited independently of each other.
Mendel’s Legacy
You might think that Mendel’s discoveries would have made a big impact on science as soon as he made them, but you would be wrong. Why? Because Mendel’s work was largely ignored. Mendel was far ahead of his time, and he was working from a remote monastery. He had no reputation in the scientific community and had only published sparingly in the past. Additionally, he published this research in an obscure scientific journal. As a result, when Charles Darwin published his landmark book on evolution in 1869, although Mendel’s work had been published just a few years earlier, Darwin was unaware of it. Consequently, Darwin knew nothing about Mendel’s laws, and didn’t understand heredity. This made Darwin’s arguments about evolution less convincing to many.
Then, in 1900, three different European scientists — Hugo de DeVries , Carl Correns , and Erich von Tschermak — arrived independently at Mendel’s laws. All three had done experiments similar to Mendel’s and come to the same conclusions that he had drawn several decades earlier. Only then was Mendel’s work rediscovered, so that Mendel himself could be given the credit he was due. Although Mendel knew nothing about genes, which were discovered after his death, he is now considered the father of genetics.
5.10 Cultural Connection
Corn is the world’s most produced crop. Canada produces 13,000-14,000 metric Kilo tonnes of corn annually, mostly in fields in Ontario, Quebec and Manitoba. Approximately 1.5 million hectares are devoted to this crop which is critically important for both humans and livestock as a food source. Despite these high numbers of output, Canada is still only 11th on the list of world corn producers, with USA, China and Brazil claiming the top three places. How did corn become such an important part of modern agriculture?
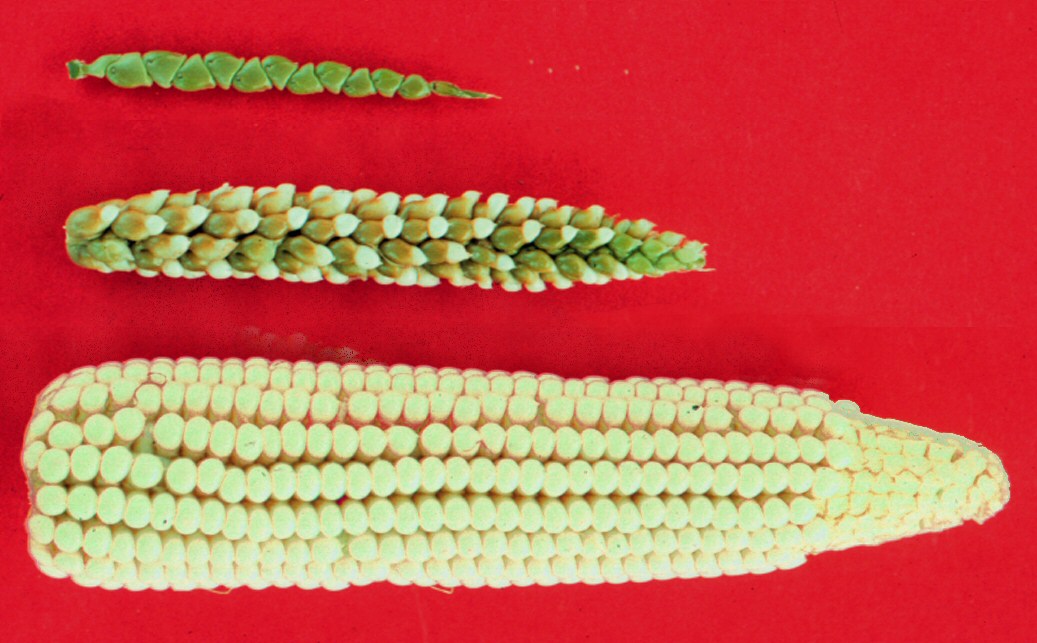
We didn’t always have corn as we know it. Modern corn is descended from a type of grass called teosinte (Figure 5.10.7) native to Mesoamerica (southern part of North America). It is estimated that Indigenous people have been harvesting corn and corn ancestors for over 9000 years. Excavations of the Xihuatoxtla Shelter in southwestern Mexico revealed our earliest evidence of domesticated corn: maize remains on tools dating back 8,700 years.
Ancient Indigenous peoples of southern Mexico developed corn from grass plants using a process we now call selective breeding , also known as artificial selection . Teosinte doesn’t resemble the corn we have today- it had only a few kernels individually encased on very hard shells, and yet today we have multiple varieties of corn with row upon row of bare kernels. This means that ancient agriculturalists among the Indigenous people of Mexico were intentionally cross-breeding strains of teosinte, and later, early maize to create plants which had more kernels, and reduced seed casings. Watch the TED Ed video in the Explore More section to see what other changes agriculturalists have made to modern-day corn.
5.10 Summary
- Mendel experimented with the inheritance of traits in pea plants at a time when the blending theory of inheritance was popular. This is the theory that offspring have a blend of the characteristics of their parents.
- Pea plants were good choices for this research, largely because they have several visible characteristics that exist in two different forms. By controlling pollination, Mendel was able to cross pea plants with different forms of the traits.
- In Mendel’s first set of experiments, he experimented with just one characteristic at a time. The results of this set of experiments led to Mendel’s first law of inheritance, called the law of segregation . This law states that there are two factors controlling a given characteristic, one of which dominates the other, and these factors separate and go to different gametes when a parent reproduces.
- In Mendel’s second set of experiments, he experimented with two characteristics at a time. The results of this set of experiments led to Mendel’s second law of inheritance, called the law of independent assortment . This law states that the factors controlling different characteristics are inherited independently of each other.
- Mendel’s work was largely ignored during his own lifetime. However, when other researchers arrived at the same laws in 1900, Mendel’s work was rediscovered, and he was given the credit he was due. He is now considered the father of genetics.
5.10 Review Questions
- Why were pea plants a good choice for Mendel’s experiments?
- How did the outcome of Mendel’s second set of experiments lead to his second law?
- Discuss the development of Mendel’s legacy.
- If Mendel’s law of independent assortment was not correct, and characteristics were always inherited together, what types of offspring do you think would have been produced by crossing plants with yellow round seeds and green wrinkled seeds? Explain your answer.
5.10 Explore More
How Mendel’s pea plants helped us understand genetics – Hortensia Jiménez Díaz, TED-Ed, 2013.
10 Strange Hybrid Fruits, Junkyboss, 2016.
The history of the world according to corn – Chris A. Kniesly, TED-Ed, 2019.

Attributions
Figure 5.10.1
Purple sweet pea flower by unknown on Yana Ray on publicdomainpictures.net is used under a CC0 1.0 p ublic domain dedication license ( https://creativecommons.org/publicdomain/zero/1.0/deed.en) .
Figure 5.10.2
Gregor_Mendel by unknown from National Institutes of Health , Health & Human Services on Wikimedia Commons is in the public domain (https://en.wikipedia.org/wiki/Public_domain).
Figure 5.10.3
Gregor Mendel in Lego by Alan on Flickr is used under a CC BY-NC-SA 2.0 (https://creativecommons.org/licenses/by-nc-sa/2.0/) license.
Figure 5.10.4
Mendels_peas by Mariana Ruiz [ LadyofHats ] on Wikimedia Commons is used under a CC0 1.0 p ublic domain dedication license ( https://creativecommons.org/publicdomain/zero/1.0/deed.en) .
Figure 5.10.5
Mendel’s first experiment with pea plants by CK-12 Foundation is used under a CC BY-NC 3.0 (https://creativecommons.org/licenses/by-nc/3.0/) license.

Figure 5.10.6
Mendel’s Second Experiment by by CK-12 Foundation is used under a CC BY-NC 3.0 (https://creativecommons.org/licenses/by-nc/3.0/) license.
Figure 5.10.7
Maize-teosinte by John Doebley on Wikimedia Commons is used under a CC BY 3.0 (https://creativecommons.org/licenses/by/3.0/deed.en) license.
Brainard, J/ CK-12 Foundation. (2016). Figure 5 Mendel’s first experiment [digital image]. In CK-12 College Human Biology (Section 5.9) [online Flexbook]. CK12.org. https://www.ck12.org/book/ck-12-human-biology/section/5.9/
Brainard, J/ CK-12 Foundation. (2016). Figure 6 Mendel’s second experiment [digital image]. In CK-12 College Human Biology (Section 5.9) [online Flexbook]. CK12.org. https://www.ck12.org/book/ck-12-human-biology/section/5.9/
Junkyboss. (2016, March 31). 10 Strange hybrid fruits. YouTube. https://www.youtube.com/watch?v=ogc367xyzfk&feature=youtu.be
TED-Ed. (2013, March 12). How Mendel’s pea plants helped us understand genetics – Hortensia Jiménez Díaz. YouTube. https://www.youtube.com/watch?v=Mehz7tCxjSE&feature=youtu.be
TED-Ed. (2019, November 26). The history of the world according to corn – Chris A. Kniesly. YouTube. https://www.youtube.com/watch?v=i6teBcfKpik&feature=youtu.be
Wikipedia contributors. (2020, June 1). Carl Correns. In Wikipedia . https://en.wikipedia.org/w/index.php?title=Carl_Correns&oldid=960172546
Wikipedia contributors. (2020, July 8). Charles Darwin. In Wikipedia. https://en.wikipedia.org/w/index.php?title=Charles_Darwin&oldid=966652322
Wikipedia contributors. (2020, March 9). Erich von Tschermak. In Wikipedia. https://en.wikipedia.org/w/index.php?title=Erich_von_Tschermak&oldid=944695823
Wikipedia contributors. (2020, July 7). Hugo de Vries. In Wikipedia . https://en.wikipedia.org/w/index.php?title=Hugo_de_Vries&oldid=966513671
The innermost layer of the hair shaft. This nearly invisible layer is the most soft and fragile, and serves as the pith or marrow of the hair.
Located between the hair cuticle and medulla and is the thickest hair layer. It also contains most of the hair's pigment, giving the hair its color. The pigment in the cortex is melanin, which is also found in skin.
When one plant pollinates a plant of another variety. The two plants' genetic material combines and the resulting seeds from that pollination will have characteristics of both varieties and is a new variety.
The outermost part of the hair shaft. It is formed from dead cells, overlapping in layers, which form scales that strengthen and protect the hair shaft.
A part of a hair that is visible above the surface of the skin and consists of dead keratinocytes.
The part of a hair that is located within the hair follicle and consists of living keratinocytes.
The identification by humans of desirable traits in plants and animals, and the steps taken to enhance and perpetuate those traits in future generations.
Biology: A Human Approach by Molly Ostwald is licensed under a Creative Commons Attribution-NonCommercial 4.0 International License , except where otherwise noted.
Share This Book
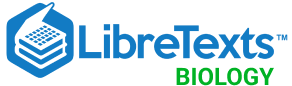
- school Campus Bookshelves
- menu_book Bookshelves
- perm_media Learning Objects
- login Login
- how_to_reg Request Instructor Account
- hub Instructor Commons
Margin Size
- Download Page (PDF)
- Download Full Book (PDF)
- Periodic Table
- Physics Constants
- Scientific Calculator
- Reference & Cite
- Tools expand_more
- Readability
selected template will load here
This action is not available.
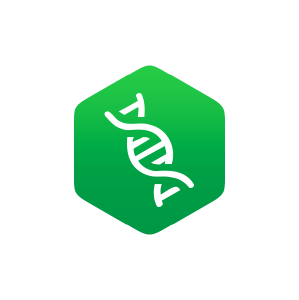
Section 4.3: Mendel’s Experiments
- Last updated
- Save as PDF
- Page ID 142562
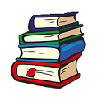
- San Francisco City College
\( \newcommand{\vecs}[1]{\overset { \scriptstyle \rightharpoonup} {\mathbf{#1}} } \)
\( \newcommand{\vecd}[1]{\overset{-\!-\!\rightharpoonup}{\vphantom{a}\smash {#1}}} \)
\( \newcommand{\id}{\mathrm{id}}\) \( \newcommand{\Span}{\mathrm{span}}\)
( \newcommand{\kernel}{\mathrm{null}\,}\) \( \newcommand{\range}{\mathrm{range}\,}\)
\( \newcommand{\RealPart}{\mathrm{Re}}\) \( \newcommand{\ImaginaryPart}{\mathrm{Im}}\)
\( \newcommand{\Argument}{\mathrm{Arg}}\) \( \newcommand{\norm}[1]{\| #1 \|}\)
\( \newcommand{\inner}[2]{\langle #1, #2 \rangle}\)
\( \newcommand{\Span}{\mathrm{span}}\)
\( \newcommand{\id}{\mathrm{id}}\)
\( \newcommand{\kernel}{\mathrm{null}\,}\)
\( \newcommand{\range}{\mathrm{range}\,}\)
\( \newcommand{\RealPart}{\mathrm{Re}}\)
\( \newcommand{\ImaginaryPart}{\mathrm{Im}}\)
\( \newcommand{\Argument}{\mathrm{Arg}}\)
\( \newcommand{\norm}[1]{\| #1 \|}\)
\( \newcommand{\Span}{\mathrm{span}}\) \( \newcommand{\AA}{\unicode[.8,0]{x212B}}\)
\( \newcommand{\vectorA}[1]{\vec{#1}} % arrow\)
\( \newcommand{\vectorAt}[1]{\vec{\text{#1}}} % arrow\)
\( \newcommand{\vectorB}[1]{\overset { \scriptstyle \rightharpoonup} {\mathbf{#1}} } \)
\( \newcommand{\vectorC}[1]{\textbf{#1}} \)
\( \newcommand{\vectorD}[1]{\overrightarrow{#1}} \)
\( \newcommand{\vectorDt}[1]{\overrightarrow{\text{#1}}} \)
\( \newcommand{\vectE}[1]{\overset{-\!-\!\rightharpoonup}{\vphantom{a}\smash{\mathbf {#1}}}} \)
Learning Objectives
- Describe the different laws of genetics developed by Mendel.
- Utilize Punnett square to perform and follow a monohybrid cross.
- Explain how pedigree trees are constructed and how to use them to study genetic diseases.
Mendel’s Crosses
Mendel’s seminal work was accomplished using the garden pea, Pisum sativum , to study inheritance. This species naturally self-fertilizes, meaning that pollen encounters ova within the same flower. The flower petals remain sealed tightly until pollination is completed to prevent the pollination of other plants. The result is highly inbred, or “ true-breeding ,” pea plants. These are plants that always produce offspring that look like the parent. By experimenting with true-breeding pea plants, Mendel avoided the appearance of unexpected traits in offspring that might occur if the plants were not true breeding. The garden pea also grows to maturity within one season, meaning that several generations could be evaluated over a relatively short time. Finally, large quantities of garden peas could be cultivated simultaneously, allowing Mendel to conclude that his results did not come about simply by chance.
Monohybrid Crosses
Mendel performed hybridizations , which involve mating two true-breeding individuals that have different traits. In the pea, which is naturally self-pollinating, this is done by manually transferring pollen from the anther of a mature pea plant of one variety to the stigma of a separate mature pea plant of the second variety.
Plants used in first-generation crosses were called P , or parental generation , plants (Figure \(\PageIndex{1}\)). Mendel collected the seeds produced by the P plants that resulted from each cross and grew them the following season. These offspring were called the F 1 , or the first filial (filial = daughter or son), generation. Once Mendel examined the characteristics in the F 1 generation of plants, he allowed them to self-fertilize naturally. He then collected and grew the seeds from the F 1 plants to produce the F 2 , or second filial , generation. Mendel’s experiments extended beyond the F 2 generation to the F 3 generation, F 4 generation, and so on, but it was the ratio of characteristics in the P, F 1 , and F 2 generations that were the most intriguing and became the basis of Mendel’s postulates.
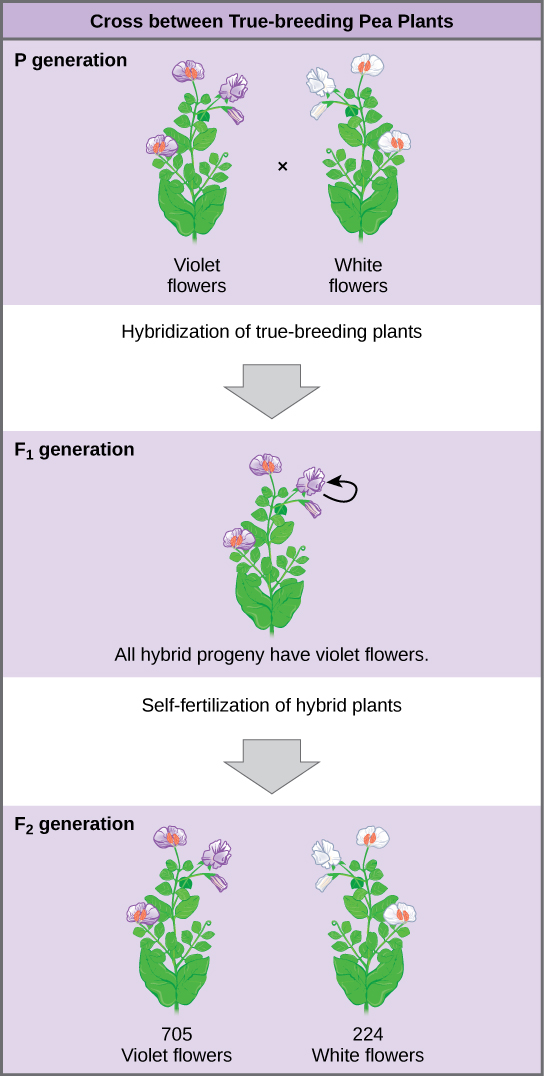
A monohybrid cross is one in which both parents are heterozygous (or a hybrid) for a single (mono) trait. The figure above shows the trait petal colour.
The ideal gas law is easy to remember and apply in solving problems, as long as you get the proper values a
Example \(\PageIndex{1}\)
Add example text here.
Definition: Term
F1 : first filial generation in a cross; the offspring of the parental generation.
F2 : second filial generation produced when F 1 individuals are self-crossed or fertilized with each other.
Hybridization : process of mating two individuals that differ with the goal of achieving a certain characteristic in their offspring.
Monohybrid : result of a cross between two true-breeding parents that express different traits for only one characteristic.
P or P0 : parental generation in a cross.
Contributors and Attributions
Samantha Fowler (Clayton State University), Rebecca Roush (Sandhills Community College), James Wise (Hampton University). Original content by OpenStax (CC BY 4.0; Access for free at https://cnx.org/contents/b3c1e1d2-83...4-e119a8aafbdd ).
Query \(\PageIndex{1}\)
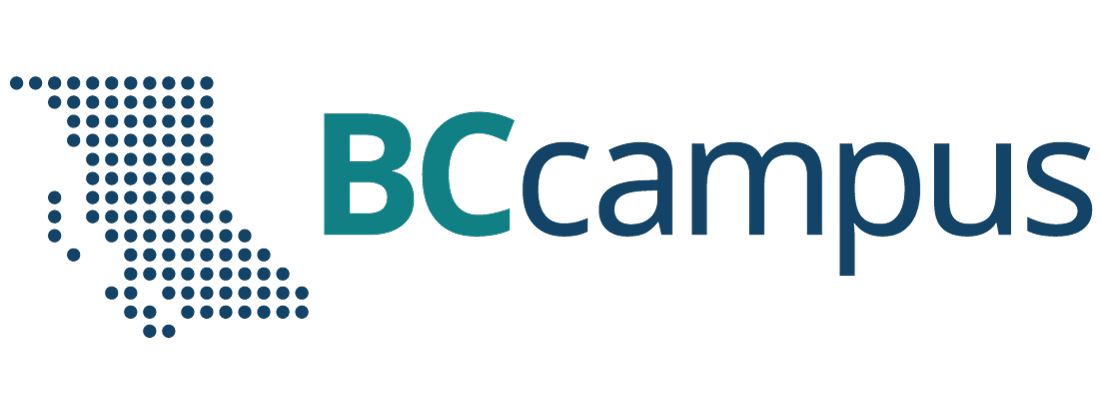
Want to create or adapt books like this? Learn more about how Pressbooks supports open publishing practices.
Chapter 8: Introduction to Patterns of Inheritance
8.1 Mendel’s Experiments
Learning objectives.
By the end of this section, you will be able to:
- Explain the scientific reasons for the success of Mendel’s experimental work
- Describe the expected outcomes of monohybrid crosses involving dominant and recessive alleles.
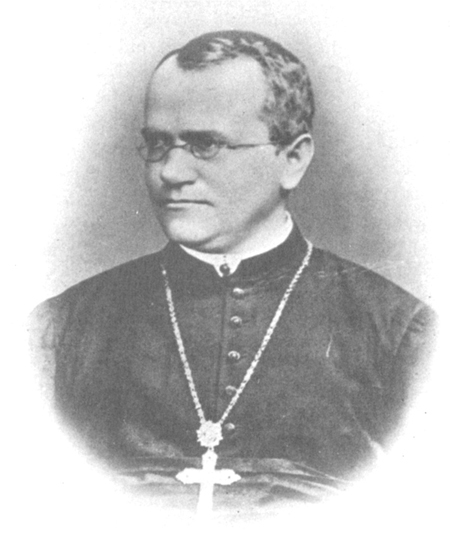
Watch the interactive video
Johann Gregor Mendel (1822–1884) was a lifelong learner, teacher, scientist, and man of faith. As a young adult, he joined the Augustinian Abbey of St. Thomas in Brno in what is now the Czech Republic. Supported by the monastery, he taught physics, botany, and natural science courses at the secondary and university levels. In 1856, he began a decade-long research pursuit involving inheritance patterns in honeybees and plants, ultimately settling on pea plants as his primary model system (a system with convenient characteristics that is used to study a specific biological phenomenon to gain understanding to be applied to other systems). In 1865, Mendel presented the results of his experiments with nearly 30,000 pea plants to the local natural history society. He demonstrated that traits are transmitted faithfully from parents to offspring in specific patterns. In 1866, he published his work, Experiments in Plant Hybridization, 1 in the proceedings of the Natural History Society of Brünn.
Mendel’s work went virtually unnoticed by the scientific community, which incorrectly believed that the process of inheritance involved a blending of parental traits that produced an intermediate physical appearance in offspring. This hypothetical process appeared to be correct because of what we know now as continuous variation. Continuous variation is the range of small differences we see among individuals in a characteristic like human height. It does appear that offspring are a “blend” of their parents’ traits when we look at characteristics that exhibit continuous variation. Mendel worked instead with traits that show discontinuous variation . Discontinuous variation is the variation seen among individuals when each individual shows one of two—or a very few—easily distinguishable traits, such as violet or white flowers. Mendel’s choice of these kinds of traits allowed him to see experimentally that the traits were not blended in the offspring as would have been expected at the time, but that they were inherited as distinct traits. In 1868, Mendel became abbot of the monastery and exchanged his scientific pursuits for his pastoral duties. He was not recognized for his extraordinary scientific contributions during his lifetime; in fact, it was not until 1900 that his work was rediscovered, reproduced, and revitalized by scientists on the brink of discovering the chromosomal basis of heredity.
Mendel’s Crosses
Mendel’s seminal work was accomplished using the garden pea , Pisum sativum , to study inheritance. This species naturally self-fertilizes , meaning that pollen encounters ova within the same flower. The flower petals remain sealed tightly until pollination is completed to prevent the pollination of other plants. The result is highly inbred, or “true-breeding,” pea plants. These are plants that always produce offspring that look like the parent. By experimenting with true-breeding pea plants, Mendel avoided the appearance of unexpected traits in offspring that might occur if the plants were not true breeding. The garden pea also grows to maturity within one season, meaning that several generations could be evaluated over a relatively short time. Finally, large quantities of garden peas could be cultivated simultaneously, allowing Mendel to conclude that his results did not come about simply by chance.
Mendel performed hybridizations, which involve mating two true-breeding individuals that have different traits. In the pea, which is naturally self-pollinating, this is done by manually transferring pollen from the anther of a mature pea plant of one variety to the stigma of a separate mature pea plant of the second variety.
Plants used in first-generation crosses were called P, or parental generation , plants ( Figure 8.3 ). Mendel collected the seeds produced by the P plants that resulted from each cross and grew them the following season. These offspring were called the F 1 , or the first filial (filial = daughter or son), generation. Once Mendel examined the characteristics in the F 1 generation of plants , he allowed them to self-fertilize naturally. He then collected and grew the seeds from the F 1 plants to produce the F 2 , or second filial, generation. Mendel’s experiments extended beyond the F 2 generation to the F 3 generation, F 4 generation, and so on, but it was the ratio of characteristics in the P, F 1 , and F 2 generations that were the most intriguing and became the basis of Mendel’s postulates.
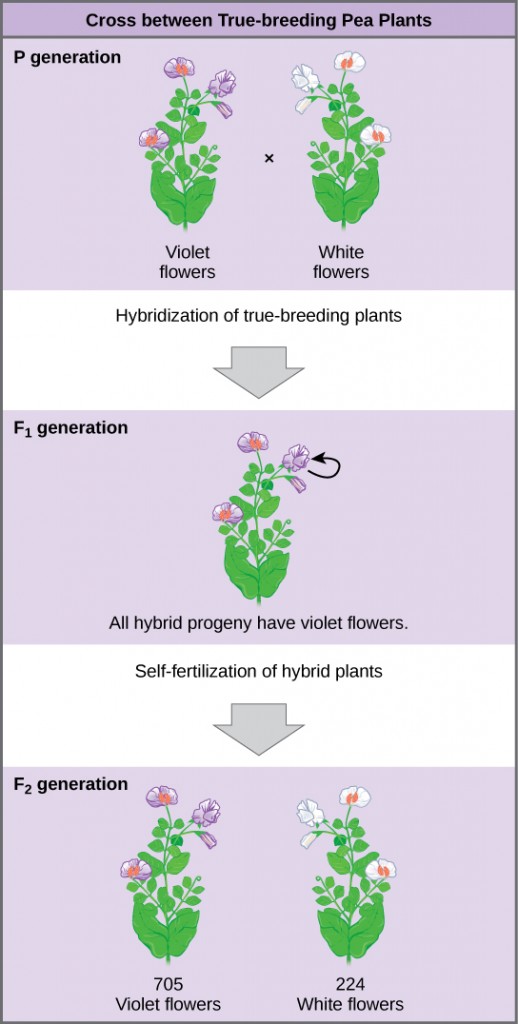
Garden Pea Characteristics Revealed the Basics of Heredity
In his 1865 publication, Mendel reported the results of his crosses involving seven different characteristics, each with two contrasting traits. A trait is defined as a variation in the physical appearance of a heritable characteristic. The characteristics included plant height, seed texture, seed color, flower color, pea-pod size, pea-pod color, and flower position. For the characteristic of flower color, for example, the two contrasting traits were white versus violet. To fully examine each characteristic, Mendel generated large numbers of F 1 and F 2 plants and reported results from thousands of F 2 plants.
What results did Mendel find in his crosses for flower color? First, Mendel confirmed that he was using plants that bred true for white or violet flower color. Irrespective of the number of generations that Mendel examined, all self-crossed offspring of parents with white flowers had white flowers, and all self-crossed offspring of parents with violet flowers had violet flowers. In addition, Mendel confirmed that, other than flower color, the pea plants were physically identical. This was an important check to make sure that the two varieties of pea plants only differed with respect to one trait, flower color.
Once these validations were complete, Mendel applied the pollen from a plant with violet flowers to the stigma of a plant with white flowers. After gathering and sowing the seeds that resulted from this cross, Mendel found that 100 percent of the F 1 hybrid generation had violet flowers. Conventional wisdom at that time would have predicted the hybrid flowers to be pale violet or for hybrid plants to have equal numbers of white and violet flowers. In other words, the contrasting parental traits were expected to blend in the offspring. Instead, Mendel’s results demonstrated that the white flower trait had completely disappeared in the F 1 generation.
Importantly, Mendel did not stop his experimentation there. He allowed the F 1 plants to self-fertilize and found that 705 plants in the F 2 generation had violet flowers and 224 had white flowers. This was a ratio of 3.15 violet flowers to one white flower, or approximately 3:1 . When Mendel transferred pollen from a plant with violet flowers to the stigma of a plant with white flowers and vice versa, he obtained approximately the same ratio irrespective of which parent—male or female—contributed which trait. This is called a reciprocal cross —a paired cross in which the respective traits of the male and female in one cross become the respective traits of the female and male in the other cross. For the other six characteristics that Mendel examined, the F 1 and F 2 generations behaved in the same way that they behaved for flower color. One of the two traits would disappear completely from the F 1 generation, only to reappear in the F 2 generation at a ratio of roughly 3:1 ( Figure 8.4 ).
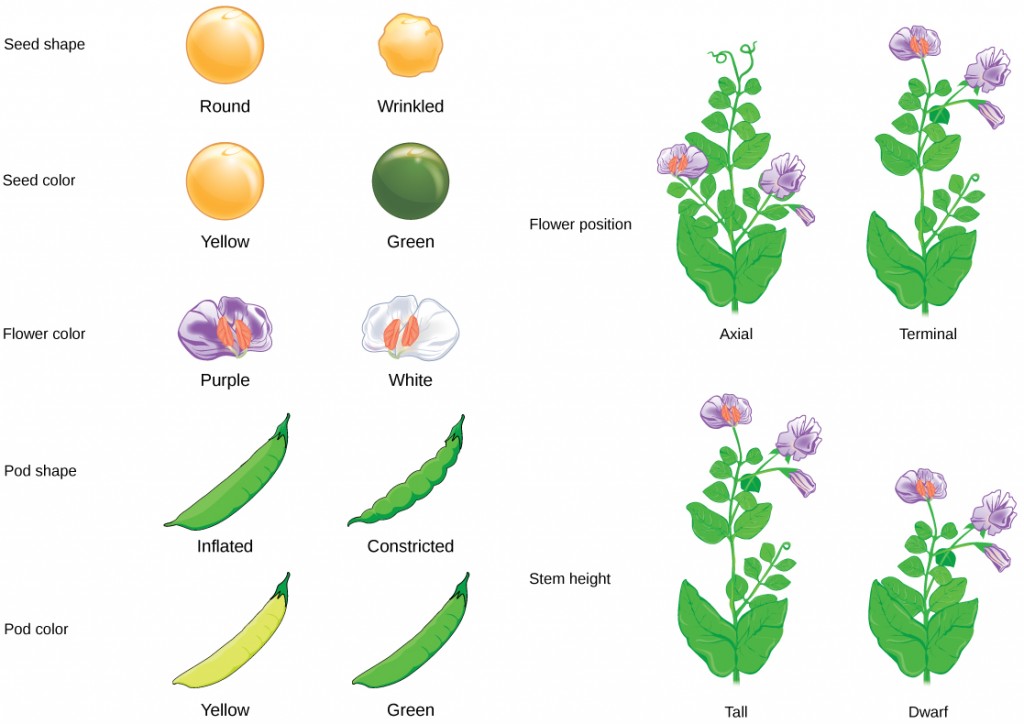
Upon compiling his results for many thousands of plants, Mendel concluded that the characteristics could be divided into expressed and latent traits. He called these dominant and recessive traits , respectively. Dominant traits are those that are inherited unchanged in a hybridization. Recessive traits become latent, or disappear in the offspring of a hybridization. The recessive trait does, however, reappear in the progeny of the hybrid offspring. An example of a dominant trait is the violet-colored flower trait. For this same characteristic (flower color), white-colored flowers are a recessive trait. The fact that the recessive trait reappeared in the F 2 generation meant that the traits remained separate (and were not blended) in the plants of the F 1 generation. Mendel proposed that this was because the plants possessed two copies of the trait for the flower-color characteristic, and that each parent transmitted one of their two copies to their offspring, where they came together. Moreover, the physical observation of a dominant trait could mean that the genetic composition of the organism included two dominant versions of the characteristic, or that it included one dominant and one recessive version. Conversely, the observation of a recessive trait meant that the organism lacked any dominant versions of this characteristic.
Concept in Action
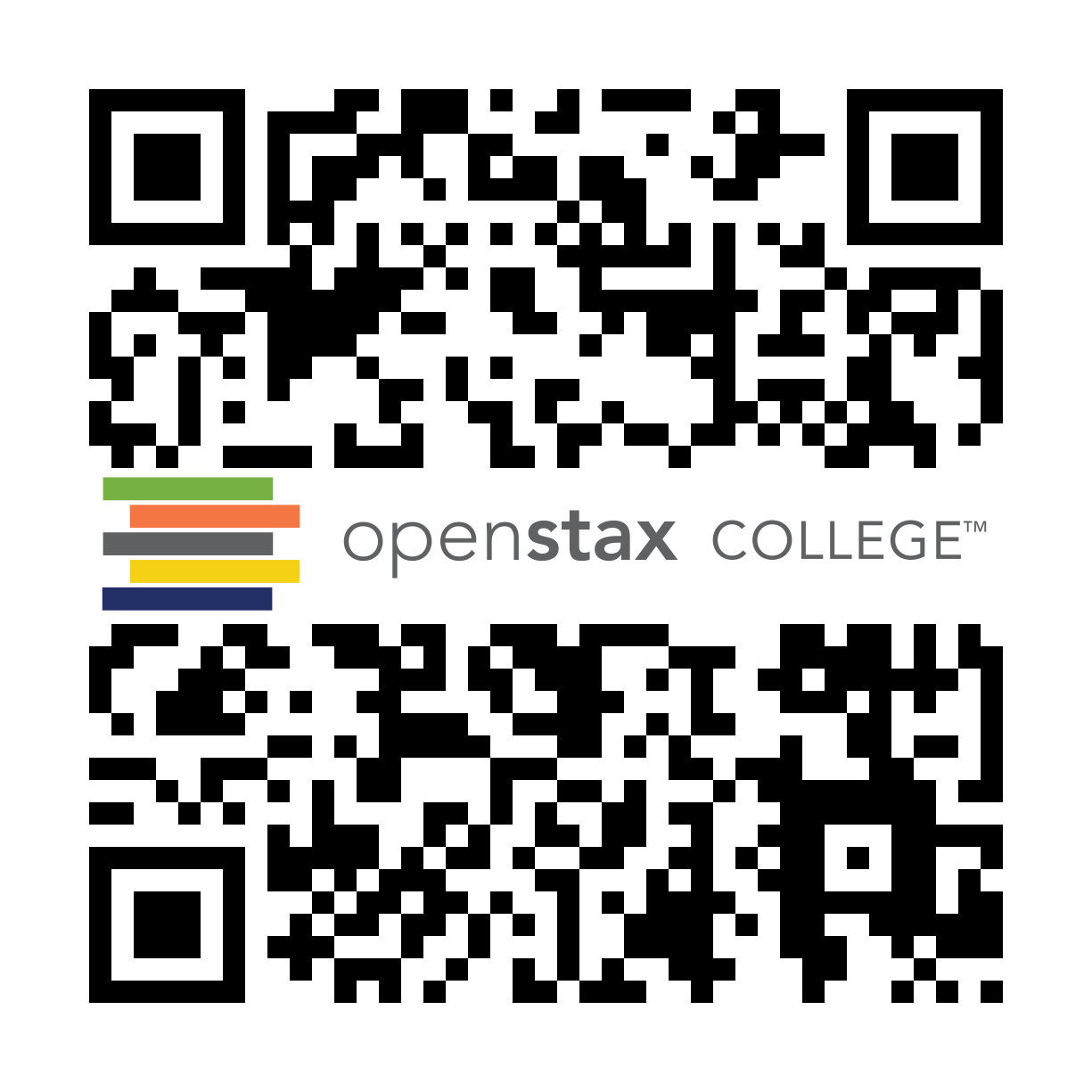
For an excellent review of Mendel’s experiments and to perform your own crosses and identify patterns of inheritance, visit the Mendel’s Peas web lab.
Section Summary
Working with garden pea plants, Mendel found that crosses between parents that differed for one trait produced F 1 offspring that all expressed one parent’s traits. The traits that were visible in the F 1 generation are referred to as dominant, and traits that disappear in the F 1 generation are described as recessive. When the F 1 plants in Mendel’s experiment were self-crossed, the F 2 offspring exhibited the dominant trait or the recessive trait in a 3:1 ratio, confirming that the recessive trait had been transmitted faithfully from the original P parent. Reciprocal crosses generated identical F 1 and F 2 offspring ratios. By examining sample sizes, Mendel showed that traits were inherited as independent events.
continuous variation: a variation in a characteristic in which individuals show a range of traits with small differences between them
discontinuous variation: a variation in a characteristic in which individuals show two, or a few, traits with large differences between them
dominant: describes a trait that masks the expression of another trait when both versions of the gene are present in an individual
F 1: the first filial generation in a cross; the offspring of the parental generation
F 2: the second filial generation produced when F 1 individuals are self-crossed or fertilized with each other
hybridization: the process of mating two individuals that differ, with the goal of achieving a certain characteristic in their offspring
model system: a species or biological system used to study a specific biological phenomenon to gain understanding that will be applied to other species
P: the parental generation in a cross
recessive: describes a trait whose expression is masked by another trait when the alleles for both traits are present in an individual
reciprocal cross: a paired cross in which the respective traits of the male and female in one cross become the respective traits of the female and male in the other cross
trait: a variation in an inherited characteristic
1 Johann Gregor Mendel, “Versuche über Pflanzenhybriden.” Verhandlungen des naturforschenden Vereines in Brünn , Bd. IV für das Jahr, 1865 Abhandlungen (1866):3–47. [for English translation, see http://www.mendelweb.org/Mendel.plain.html]
Concepts of Biology - 1st Canadian Edition Copyright © 2015 by Charles Molnar and Jane Gair is licensed under a Creative Commons Attribution 4.0 International License , except where otherwise noted.
Share This Book
12. Mendel’s Experiments and Heredity
Mendel’s experiments and the laws of probability, learning objectives.
By the end of this section, you will be able to do the following:
- Describe the scientific reasons for the success of Mendel’s experimental work
- Describe the expected outcomes of monohybrid crosses involving dominant and recessive alleles
- Apply the sum and product rules to calculate probabilities
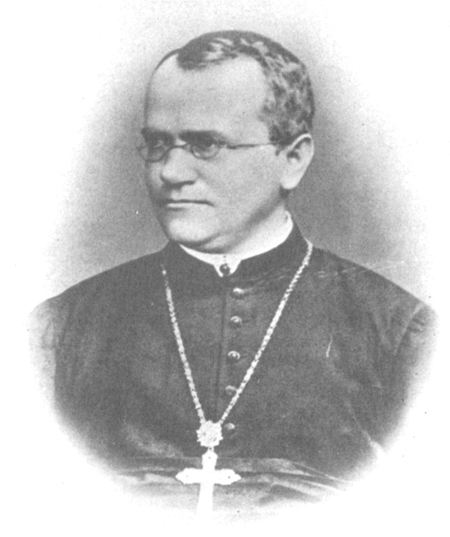
Johann Gregor Mendel (1822–1884) ( (Figure) ) was a lifelong learner, teacher, scientist, and man of faith. As a young adult, he joined the Augustinian Abbey of St. Thomas in Brno in what is now the Czech Republic. Supported by the monastery, he taught physics, botany, and natural science courses at the secondary and university levels. In 1856, he began a decade-long research pursuit involving inheritance patterns in honeybees and plants, ultimately settling on pea plants as his primary model system (a system with convenient characteristics used to study a specific biological phenomenon to be applied to other systems). In 1865, Mendel presented the results of his experiments with nearly 30,000 pea plants to the local Natural History Society. He demonstrated that traits are transmitted from parents to offspring independently of other traits and in dominant and recessive patterns. In 1866, he published his work, Experiments in Plant Hybridization, 1 in the proceedings of the Natural History Society of Brünn.
Mendel’s work went virtually unnoticed by the scientific community, which believed, incorrectly, that the process of inheritance involved a blending of parental traits that produced an intermediate physical appearance in offspring. The blending theory of inheritance asserted that the original parental traits were lost or absorbed by the blending in the offspring, but we now know that this is not the case. This hypothetical process appeared to be correct because of what we know now as continuous variation. Continuous variation results from the action of many genes to determine a characteristic like human height. Offspring appear to be a “blend” of their parents’ traits.
Instead of continuous characteristics, Mendel worked with traits that were inherited in distinct classes (specifically, violet versus white flowers); this is referred to as discontinuous variation. Mendel’s choice of these kinds of traits allowed him to see experimentally that the traits were not blended in the offspring, nor were they absorbed, but rather that they kept their distinctness and could be passed on. In 1868, Mendel became abbot of the monastery and exchanged his scientific pursuits for his pastoral duties. He was not recognized for his extraordinary scientific contributions during his lifetime. In fact, it was not until 1900 that his work was rediscovered, reproduced, and revitalized by scientists on the brink of discovering the chromosomal basis of heredity.
Mendel’s Model System
Mendel’s seminal work was accomplished using the garden pea, Pisum sativum , to study inheritance. This species naturally self-fertilizes, such that pollen encounters ova within individual flowers. The flower petals remain sealed tightly until after pollination, preventing pollination from other plants. The result is highly inbred, or “true-breeding,” pea plants. These are plants that always produce offspring that look like the parent. By experimenting with true-breeding pea plants, Mendel avoided the appearance of unexpected traits in offspring that might occur if the plants were not true breeding. The garden pea also grows to maturity within one season, meaning that several generations could be evaluated over a relatively short time. Finally, large quantities of garden peas could be cultivated simultaneously, allowing Mendel to conclude that his results did not come about simply by chance.
Mendelian Crosses
Mendel performed hybridizations, which involve mating two true-breeding individuals that have different traits. In the pea, which is naturally self-pollinating, this is done by manually transferring pollen from the anther of a mature pea plant of one variety to the stigma of a separate mature pea plant of the second variety. In plants, pollen carries the male gametes (sperm) to the stigma, a sticky organ that traps pollen and allows the sperm to move down the pistil to the female gametes (ova) below. To prevent the pea plant that was receiving pollen from self-fertilizing and confounding his results, Mendel painstakingly removed all of the anthers from the plant’s flowers before they had a chance to mature.
Plants used in first-generation crosses were called P 0 , or parental generation one ( (Figure) ). After each cross, Mendel collected the seeds belonging to the P 0 plants and grew them the following season. These offspring were called the F 1 , or the first filial ( filial = offspring, daughter or son) generation. Once Mendel examined the characteristics in the F 1 generation of plants, he allowed them to self-fertilize naturally. He then collected and grew the seeds from the F 1 plants to produce the F 2 , or second filial, generation. Mendel’s experiments extended beyond the F 2 generation to the F 3 and F 4 generations, and so on, but it was the ratio of characteristics in the P 0 −F 1 −F 2 generations that were the most intriguing and became the basis for Mendel’s postulates.
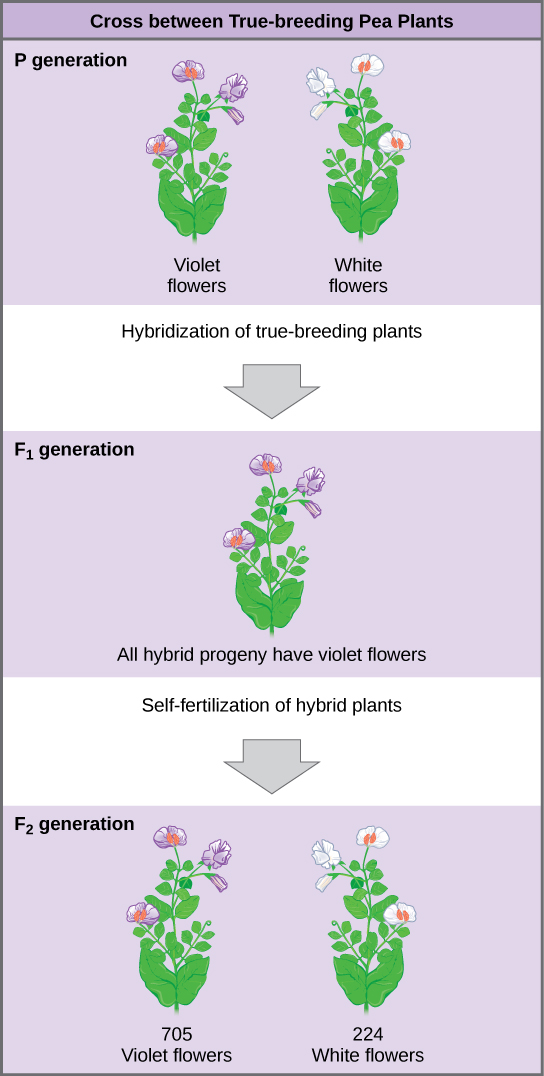
Garden Pea Characteristics Revealed the Basics of Heredity
In his 1865 publication, Mendel reported the results of his crosses involving seven different characteristics, each with two contrasting traits. A trait is defined as a variation in the physical appearance of a heritable characteristic. The characteristics included plant height, seed texture, seed color, flower color, pea pod size, pea pod color, and flower position. For the characteristic of flower color, for example, the two contrasting traits were white versus violet. To fully examine each characteristic, Mendel generated large numbers of F 1 and F 2 plants, reporting results from 19,959 F 2 plants alone. His findings were consistent.
What results did Mendel find in his crosses for flower color? First, Mendel confirmed that he had plants that bred true for white or violet flower color. Regardless of how many generations Mendel examined, all self-crossed offspring of parents with white flowers had white flowers, and all self-crossed offspring of parents with violet flowers had violet flowers. In addition, Mendel confirmed that, other than flower color, the pea plants were physically identical.
Once these validations were complete, Mendel applied the pollen from a plant with violet flowers to the stigma of a plant with white flowers. After gathering and sowing the seeds that resulted from this cross, Mendel found that 100 percent of the F 1 hybrid generation had violet flowers . Conventional wisdom at that time (the blending theory) would have predicted the hybrid flowers to be pale violet or for hybrid plants to have equal numbers of white and violet flowers. In other words, the contrasting parental traits were expected to blend in the offspring. Instead, Mendel’s results demonstrated that the white flower trait in the F 1 generation had completely disappeared.
Importantly, Mendel did not stop his experimentation there. He allowed the F 1 plants to self-fertilize and found that, of F 2 -generation plants, 705 had violet flowers and 224 had white flowers. This was a ratio of 3.15 violet flowers per one white flower, or approximately 3:1. When Mendel transferred pollen from a plant with violet flowers to the stigma of a plant with white flowers and vice versa, he obtained about the same ratio regardless of which parent, male or female, contributed which trait. This is called a reciprocal cross—a paired cross in which the respective traits of the male and female in one cross become the respective traits of the female and male in the other cross. For the other six characteristics Mendel examined, the F 1 and F 2 generations behaved in the same way as they had for flower color. One of the two traits would disappear completely from the F 1 generation only to reappear in the F 2 generation at a ratio of approximately 3:1 ( (Figure) ).
Upon compiling his results for many thousands of plants, Mendel concluded that the characteristics could be divided into expressed and latent traits. He called these, respectively, dominant and recessive traits. Dominant traits are those that are inherited unchanged in a hybridization. Recessive traits become latent, or disappear, in the offspring of a hybridization. The recessive trait does, however, reappear in the progeny of the hybrid offspring. An example of a dominant trait is the violet-flower trait. For this same characteristic (flower color), white-colored flowers are a recessive trait. The fact that the recessive trait reappeared in the F 2 generation meant that the traits remained separate (not blended) in the plants of the F 1 generation. Mendel also proposed that plants possessed two copies of the trait for the flower-color characteristic, and that each parent transmitted one of its two copies to its offspring, where they came together. Moreover, the physical observation of a dominant trait could mean that the genetic composition of the organism included two dominant versions of the characteristic or that it included one dominant and one recessive version. Conversely, the observation of a recessive trait meant that the organism lacked any dominant versions of this characteristic.
So why did Mendel repeatedly obtain 3:1 ratios in his crosses? To understand how Mendel deduced the basic mechanisms of inheritance that lead to such ratios, we must first review the laws of probability.
Probability Basics
Probabilities are mathematical measures of likelihood. The empirical probability of an event is calculated by dividing the number of times the event occurs by the total number of opportunities for the event to occur. It is also possible to calculate theoretical probabilities by dividing the number of times that an event is expected to occur by the number of times that it could occur. Empirical probabilities come from observations, like those of Mendel. Theoretical probabilities, on the other hand, come from knowing how the events are produced and assuming that the probabilities of individual outcomes are equal. A probability of one for some event indicates that it is guaranteed to occur, whereas a probability of zero indicates that it is guaranteed not to occur. An example of a genetic event is a round seed produced by a pea plant.
In one experiment, Mendel demonstrated that the probability of the event “round seed” occurring was one in the F 1 offspring of true-breeding parents, one of which has round seeds and one of which has wrinkled seeds. When the F 1 plants were subsequently self-crossed, the probability of any given F 2 offspring having round seeds was now three out of four. In other words, in a large population of F 2 offspring chosen at random, 75 percent were expected to have round seeds, whereas 25 percent were expected to have wrinkled seeds. Using large numbers of crosses, Mendel was able to calculate probabilities and use these to predict the outcomes of other crosses.
The Product Rule and Sum Rule
Mendel demonstrated that pea plants transmit characteristics as discrete units from parent to offspring. As will be discussed, Mendel also determined that different characteristics, like seed color and seed texture, were transmitted independently of one another and could be considered in separate probability analyses. For instance, performing a cross between a plant with green, wrinkled seeds and a plant with yellow, round seeds still produced offspring that had a 3:1 ratio of green:yellow seeds (ignoring seed texture) and a 3:1 ratio of round:wrinkled seeds (ignoring seed color). The characteristics of color and texture did not influence each other.
The product rule of probability can be applied to this phenomenon of the independent transmission of characteristics. The product rule states that the probability of two independent events occurring together can be calculated by multiplying the individual probabilities of each event occurring alone. To demonstrate the product rule, imagine that you are rolling a six-sided die (D) and flipping a penny (P) at the same time. The die may roll any number from 1–6 (D # ), whereas the penny may turn up heads (P H ) or tails (P T ). The outcome of rolling the die has no effect on the outcome of flipping the penny and vice versa. There are 12 possible outcomes of this action ( (Figure) ), and each event is expected to occur with equal probability.
Of the 12 possible outcomes, the die has a 2/12 (or 1/6) probability of rolling a two, and the penny has a 6/12 (or 1/2) probability of coming up heads. By the product rule, the probability that you will obtain the combined outcome 2 and heads is: (D 2 ) x (P H ) = (1/6) x (1/2) or 1/12 ( (Figure) ). Notice the word “and” in the description of the probability. The “and” is a signal to apply the product rule. For example, consider how the product rule is applied to the dihybrid cross: the probability of having both dominant traits in the F 2 progeny is the product of the probabilities of having the dominant trait for each characteristic, as shown here:
On the other hand, the sum rule of probability is applied when considering two mutually exclusive outcomes that can come about by more than one pathway. The sum rule states that the probability of the occurrence of one event or the other event, of two mutually exclusive events, is the sum of their individual probabilities. Notice the word “or” in the description of the probability. The “or” indicates that you should apply the sum rule. In this case, let’s imagine you are flipping a penny (P) and a quarter (Q). What is the probability of one coin coming up heads and one coin coming up tails? This outcome can be achieved by two cases: the penny may be heads (P H ) and the quarter may be tails (Q T ), or the quarter may be heads (Q H ) and the penny may be tails (P T ). Either case fulfills the outcome. By the sum rule, we calculate the probability of obtaining one head and one tail as [(P H ) × (Q T )] + [(Q H ) × (P T )] = [(1/2) × (1/2)] + [(1/2) × (1/2)] = 1/2 ( (Figure) ). You should also notice that we used the product rule to calculate the probability of P H and Q T , and also the probability of P T and Q H , before we summed them. Again, the sum rule can be applied to show the probability of having just one dominant trait in the F 2 generation of a dihybrid cross:
To use probability laws in practice, we must work with large sample sizes because small sample sizes are prone to deviations caused by chance. The large quantities of pea plants that Mendel examined allowed him calculate the probabilities of the traits appearing in his F 2 generation. As you will learn, this discovery meant that when parental traits were known, the offspring’s traits could be predicted accurately even before fertilization.
Section Summary
Working with garden pea plants, Mendel found that crosses between parents that differed by one trait produced F 1 offspring that all expressed the traits of one parent. Observable traits are referred to as dominant, and non-expressed traits are described as recessive. When the offspring in Mendel’s experiment were self-crossed, the F 2 offspring exhibited the dominant trait or the recessive trait in a 3:1 ratio, confirming that the recessive trait had been transmitted faithfully from the original P 0 parent. Reciprocal crosses generated identical F 1 and F 2 offspring ratios. By examining sample sizes, Mendel showed that his crosses behaved reproducibly according to the laws of probability, and that the traits were inherited as independent events.
Two rules in probability can be used to find the expected proportions of offspring of different traits from different crosses. To find the probability of two or more independent events occurring together, apply the product rule and multiply the probabilities of the individual events. The use of the word “and” suggests the appropriate application of the product rule. To find the probability of two or more events occurring in combination, apply the sum rule and add their individual probabilities together. The use of the word “or” suggests the appropriate application of the sum rule.
Review Questions
Mendel performed hybridizations by transferring pollen from the _______ of the male plant to the female ova.
Show Solution
Which is one of the seven characteristics that Mendel observed in pea plants?
- flower size
- seed texture
Imagine you are performing a cross involving seed color in garden pea plants. What F 1 offspring would you expect if you cross true-breeding parents with green seeds and yellow seeds? Yellow seed color is dominant over green.
- 100 percent yellow-green seeds
- 100 percent yellow seeds
- 50 percent yellow, 50 percent green seeds
- 25 percent green, 75 percent yellow seeds
Consider a cross to investigate the pea pod texture trait, involving constricted or inflated pods. Mendel found that the traits behave according to a dominant/recessive pattern in which inflated pods were dominant. If you performed this cross and obtained 650 inflated-pod plants in the F 2 generation, approximately how many constricted-pod plants would you expect to have?
A scientist pollinates a true-breeding pea plant with violet, terminal flowers with pollen from a true-breeding pea plant with white, axial flowers. Which of the following observations would most accurately describe the F 2 generation?
- 75% violet flowers; 75% terminal flowers
- 75% white flowers in a terminal position
- 75% violet flowers; 75% axial flowers
- 75% violet flowers in an axial position
Free Response
Describe one of the reasons why the garden pea was an excellent choice of model system for studying inheritance.
The garden pea is sessile and has flowers that close tightly during self-pollination. These features help to prevent accidental or unintentional fertilizations that could have diminished the accuracy of Mendel’s data.
How would you perform a reciprocal cross for the characteristic of stem height in the garden pea?
Two sets of P 0 parents would be used. In the first cross, pollen would be transferred from a true-breeding tall plant to the stigma of a true-breeding dwarf plant. In the second cross, pollen would be transferred from a true-breeding dwarf plant to the stigma of a true-breeding tall plant. For each cross, F 1 and F 2 offspring would be analyzed to determine if offspring traits were affected according to which parent donated each trait.
Mendel performs a cross using a true-breeding pea plant with round, yellow seeds and a true-breeding pea plant with green, wrinkled seeds. What is the probability that offspring will have green, round seeds? Calculate the probability for the F 1 and F 2 generations.
Since we are calculating the probability of two independent events occurring simultaneously, we use the product rule.
F 1 generation: Since green seed color is recessive, there is a 0% probability that any plants in the F 1 generation will have green, round seeds.
F 2 generation: The probability of growing an F 2 generation plant with green seeds is ¼, while the probability of growing an F 2 generation plant with round seeds is ¾. We can use the product rule to then calculate the probability of a plant with green, round seeds:
Calculate the probability of selecting a heart or a face card from a standard deck of cards. Is this outcome more or less likely than selecting a heart suit face card?
A standard deck of cards contains 52 cards, 13 of which are hearts and 12 of which are face cards.
Heart suit or face card: This calculation requires the sum rule since there are multiple pathways to successfully pulling a desired card.
The probability of selecting a heart suit or a face card is significantly more likely than the probability of selecting a heart suit face card ([latex]3/52=5.8%[/latex]).
- 1 Johann Gregor Mendel, Versuche über Pflanzenhybriden Verhandlungen des naturforschenden Vereines in Brünn, Bd. IV für das Jahr , 1865 Abhandlungen, 3–47. [for English translation see http://www.mendelweb.org/Mendel.plain.html ]
Candela Citations
- Biology 2e. Provided by : OpenStax. Located at : https://openstax.org/details/books/biology-2e . License : CC BY: Attribution . License Terms : Download for free at http://cnx.org/contents/[email protected]
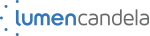
Privacy Policy
Want to create or adapt books like this? Learn more about how Pressbooks supports open publishing practices.
Mendel’s Experiments
Learning objectives.
- Explain the scientific reasons for the success of Mendel’s experimental work
- Describe the expected outcomes of monohybrid crosses involving dominant and recessive alleles
Johann Gregor Mendel (1822–1884) ( Figure 9.2 ) was a lifelong learner, teacher, scientist, and man of faith. As a young adult, he joined the Augustinian Abbey of St. Thomas in Brno in what is now the Czech Republic. Supported by the monastery, he taught physics, botany, and natural science courses at the secondary and university levels. In 1856, he began a decade-long research pursuit involving inheritance patterns in honeybees and plants, ultimately settling on pea plants as his primary model system (a system with convenient characteristics that is used to study a specific biological phenomenon to gain understanding to be applied to other systems). In 1865, Mendel presented the results of his experiments with nearly 30,000 pea plants to the local natural history society. He demonstrated that traits are transmitted faithfully from parents to offspring in specific patterns. In 1866, he published his work, Experiments in Plant Hybridization, 1 in the proceedings of the Natural History Society of Brünn. As stated earlier, in genetics, “parent” is often used to describe the individual organism(s) that contribute genetic material to an offspring, usually in the form of gamete cells.
Mendel’s work went virtually unnoticed by the scientific community, which incorrectly believed that the process of inheritance involved a blending of parental traits that produced an intermediate physical appearance in offspring. This hypothetical process appeared to them be correct because of what we know now as continuous variation. Continuous variation is the range of small differences we see among individuals in a characteristic like human height. It does appear that offspring are a “blend” of their parents’ traits when we look at characteristics that exhibit continuous variation. Mendel worked instead with traits that show discontinuous variation . Discontinuous variation is the variation seen among individuals when each individual shows one of two—or a very few—easily distinguishable traits, such as violet or white flowers. Mendel’s choice of these kinds of traits allowed him to see experimentally that the traits were not blended in the offspring as would have been expected at the time, but that they were inherited as distinct traits. In 1868, Mendel became abbot of the monastery and exchanged his scientific pursuits for his pastoral duties. He was not recognized for his extraordinary scientific contributions during his lifetime; in fact, it was not until 1900 that his work was rediscovered, reproduced, and revitalized by scientists on the brink of discovering the chromosomal basis of heredity.
Mendel’s Crosses
Mendel’s seminal work was accomplished using the garden pea, Pisum sativum , to study inheritance. This species naturally self-fertilizes, meaning that pollen encounters ova within the same flower. Because every pea plant has both male reproductive organs and female reproductive organs, each plant produces both types of gametes required for reproduction—both pollen and ova. In plants, just as in animals, reproductive organs are classified by the size of the gametes produced. The organs producing the smaller pollen are called male reproductive organs, while the organs producing the larger ova are called female reproductive organs.
In garden peas, the flower petals remain sealed tightly until pollination is completed to prevent the pollination of other plants. The result is highly inbred, or “true-breeding,” pea plants. These are plants that always produce offspring that look like the parent. By experimenting with true-breeding pea plants, Mendel avoided the appearance of unexpected traits in offspring that might occur if the plants were not true-breeding. The garden pea also grows to maturity within one season, meaning that several generations could be evaluated over a relatively short time. Finally, large quantities of garden peas could be cultivated simultaneously, allowing Mendel to conclude that his results did not come about simply by chance.
Mendel performed hybridizations , which involve mating two true-breeding individuals that have different traits. In the pea, which is naturally self-pollinating, this is done by manually transferring pollen from the anther of a mature pea plant of one variety to the stigma of a separate mature pea plant of the second variety.
Plants used in first-generation crosses were called P, or parental generation, plants ( Figure 9.3 ). Mendel collected the seeds produced by the P plants that resulted from each cross and grew them the following season. These offspring were called the F 1 , or the first filial (filial = daughter or son), generation. Once Mendel examined the characteristics in the F 1 generation of plants, he allowed them to self-fertilize naturally. He then collected and grew the seeds from the F 1 plants to produce the F 2 , or second filial, generation. Mendel’s experiments extended beyond the F 2 generation to the F 3 generation, F 4 generation, and so on, but it was the ratio of characteristics in the P, F 1 , and F 2 generations that were the most intriguing and became the basis of Mendel’s postulates.
Garden Pea Characteristics Revealed the Basics of Heredity
In his 1865 publication, Mendel reported the results of his crosses involving seven different characteristics, each with two contrasting traits. A trait is defined as a variation in the physical appearance of a heritable characteristic. The characteristics included plant height, seed texture, seed color, flower color, pea-pod size, pea-pod color, and flower position. For the characteristic of flower color, for example, the two contrasting traits were white versus violet. To fully examine each characteristic, Mendel generated large numbers of F 1 and F 2 plants and reported results from thousands of F 2 plants.
What results did Mendel find in his crosses for flower color? First, Mendel confirmed that he was using plants that bred true for white or violet flower color. Irrespective of the number of generations that Mendel examined, all self-crossed offspring of parents with white flowers had white flowers, and all self-crossed offspring of parents with violet flowers had violet flowers. In addition, Mendel confirmed that, other than flower color, the pea plants were physically identical. This was an important check to make sure that the two varieties of pea plants only differed with respect to one trait, flower color.
Once these validations were complete, Mendel applied the pollen from a plant with violet flowers to the stigma of a plant with white flowers. After gathering and sowing the seeds that resulted from this cross, Mendel found that 100 percent of the F 1 hybrid generation had violet flowers. Conventional wisdom at that time would have predicted the hybrid flowers to be pale violet or for hybrid plants to have equal numbers of white and violet flowers. In other words, the contrasting parental traits were expected to blend in the offspring. Instead, Mendel’s results demonstrated that the white flower trait had completely disappeared in the F 1 generation.
Importantly, Mendel did not stop his experimentation there. He allowed the F 1 plants to self-fertilize and found that 705 plants in the F 2 generation had violet flowers and 224 had white flowers. This was a ratio of 3.15 violet flowers to one white flower, or approximately 3:1. Mendel performed an additional experiment to ascertain differences in inheritance of traits carried in the pollen versus the ovum. When Mendel transferred pollen from a plant with violet flowers to fertilize the ova of a plant with white flowers and vice versa, he obtained approximately the same ratio irrespective of which gamete contributed which trait. This is called a reciprocal cross —a paired cross in which the respective traits of the male and female in one cross become the respective traits of the female and male in the other cross. So, in simpler terms, it didn’t matter which parent plant—the “mom” or the “dad”—had which color. The results were the same either way. Reciprocal cross is basically just a fancy way to say that swapping the roles of the male and female plants doesn’t change the outcome. For the other six characteristics that Mendel examined, the F 1 and F 2 generations behaved in the same way that they behaved for flower color. One of the two traits would disappear completely from the F 1 generation, only to reappear in the F 2 generation at a ratio of roughly 3:1 ( Figure 9.4 ).
Upon compiling his results for many thousands of plants, Mendel concluded that the characteristics could be divided into expressed and latent traits. He called these dominant and recessive traits, respectively. Dominant traits are those that are inherited unchanged in a hybridization. Recessive traits become latent, or disappear in the offspring of a hybridization. The recessive trait does, however, reappear in the progeny of the hybrid offspring. An example of a dominant trait is the violet-colored flower trait. For this same characteristic (flower color), white-colored flowers are a recessive trait. The fact that the recessive trait reappeared in the F 2 generation meant that the traits remained separate (and were not blended) in the plants of the F 1 generation. Mendel proposed that this was because the plants possessed two copies of the trait for the flower-color characteristic, and that each parent transmitted one of their two copies to their offspring, where they came together. Moreover, the physical observation of a dominant trait could mean that the genetic composition of the organism included two dominant versions of the characteristic, or that it included one dominant and one recessive version. Conversely, the observation of a recessive trait expressed outwardly meant that the organism lacked any dominant versions of this characteristic.
- 1 Johann Gregor Mendel, “Versuche über Pflanzenhybriden.” Verhandlungen des naturforschenden Vereines in Brünn , Bd. IV für das Jahr, 1865 Abhandlungen (1866):3–47. [for English translation, see http://www.mendelweb.org/Mendel.plain.html]
© OpenStax. Textbook content produced by OpenStax is licensed under a Creative Commons Attribution License. https://openstax.org/books/concepts-biology/pages/1-introduction
Introduction to Living Systems Copyright © by Dr. Becki Brunelli. All Rights Reserved.

IMAGES
COMMENTS
Mendel’s experiments extended beyond the F 2 generation to the F 3 generation, F 4 generation, and so on, but it was the ratio of characteristics in the P, F 1, and F 2 generations that were the most intriguing and became the basis of Mendel’s postulates. Figure 2: Mendel’s process for performing crosses included examining flower color.
Mendel also experimented to see what would happen if plants with 2 or more pure-bred traits were cross-bred. He found that each trait was inherited independently of the other and produced its own 3:1 ratio. This is the principle of independent assortment. Find out more about Mendel’s principles of inheritance. The next generations
Sep 22, 2021 · Mendel’s experiments extended beyond the F 2 generation to the F 3 generation, F 4 generation, and so on, but it was the ratio of characteristics in the P, F 1, and F 2 generations that were the most intriguing and became the basis of Mendel’s postulates. Figure \(\PageIndex{2}\): Mendel’s process for performing crosses included examining ...
In Mendel’s second set of experiments, he experimented with two characteristics at a time. The results of this set of experiments led to Mendel’s second law of inheritance, called the law of independent assortment. This law states that the factors controlling different characteristics are inherited independently of each other.
May 13, 2020 · Then, in 1900, three different European scientists — named DeVries, Correns, and Tschermak — independently arrived at Mendel's laws. All three had done experiments similar to Mendel's and come to the same conclusions that he had drawn several decades earlier. Only then was Mendel's work rediscovered and Mendel himself given the credit he ...
Sep 17, 2024 · Mendel’s experiments extended beyond the F 2 generation to the F 3 generation, F 4 generation, and so on, but it was the ratio of characteristics in the P, F 1, and F 2 generations that were the most intriguing and became the basis of Mendel’s postulates. Figure \(\PageIndex{1}\): Mendel’s process for performing crosses included examining ...
Mendel’s experiments extended beyond the F 2 generation to the F 3 generation, F 4 generation, and so on, but it was the ratio of characteristics in the P, F 1, and F 2 generations that were the most intriguing and became the basis of Mendel’s postulates. Figure 8.3 Mendel’s process for performing crosses included examining flower color.
Mendel’s experiments extended beyond the F 2 generation to the F 3 and F 4 generations, and so on, but it was the ratio of characteristics in the P 0 −F 1 −F 2 generations that were the most intriguing and became the basis for Mendel’s postulates.
[46] [47] Modern genetics shows that Mendelian heredity is, in fact, an inherently biological process, though not all genes of Mendel's experiments are yet understood. [48] [49] Ultimately, the two approaches were combined, especially by work conducted by R. A. Fisher as early as 1918.
Mendel’s work went virtually unnoticed by the scientific community, which incorrectly believed that the process of inheritance involved a blending of parental traits that produced an intermediate physical appearance in offspring. This hypothetical process appeared to them be correct because of what we know now as continuous variation.