- Bipolar Disorder
- Therapy Center
- When To See a Therapist
- Types of Therapy
- Best Online Therapy
- Best Couples Therapy
- Managing Stress
- Sleep and Dreaming
- Understanding Emotions
- Self-Improvement
- Healthy Relationships
- Student Resources
- Personality Types
- Guided Meditations
- Verywell Mind Insights
- 2024 Verywell Mind 25
- Mental Health in the Classroom
- Editorial Process
- Meet Our Review Board
- Crisis Support

What Is Sensory Memory?
Kendra Cherry, MS, is a psychosocial rehabilitation specialist, psychology educator, and author of the "Everything Psychology Book."
:max_bytes(150000):strip_icc():format(webp)/IMG_9791-89504ab694d54b66bbd72cb84ffb860e.jpg)
Shaheen Lakhan, MD, PhD, is an award-winning physician-scientist and clinical development specialist.
:max_bytes(150000):strip_icc():format(webp)/Shaheen-Lakhan-660-594fd00c787a40e2bcf15a60eaf9b89a.jpg)
- Types of Sensory Memory
- Sensory Memory
Characteristics of Sensory Memory
Examples of sensory memory.
- Sperling's Memory Experiments
Sensory memory is a very brief memory that allows people to retain impressions of sensory information after the original stimulus has ceased. It is often thought of as the first stage of memory that involves registering a tremendous amount of information about the environment, but only for a very brief period.
The purpose of sensory memory is to retain information long enough for it to be recognized.
At a Glance
Sensory memory is a very short-term storage for information from the senses. It persists briefly and allows for processing even after the stimulus has ended. It allows you to retain brief impressions of information so you can interpret and interact with the world around you. In some instances, this information may be transferred to short-term memory, but in most cases, it quickly fades. While sensory memory may be brief, it is crucial to attention and memory.
Types of Sensory Memory
Experts believe that different senses have different types of sensory memory. The different types of sensory memory have also been shown to have slightly different durations.
Iconic Memory
Also known as visual sensory memory, iconic memory involves a very brief image. This type of sensory memory typically lasts for about one-quarter to one-half of a second .
Echoic Memory
Also known as auditory sensory memory, echoic memory involves a very brief memory of sound a bit like an echo. This type of sensory memory can last for up to three to four seconds .
Haptic Memory
Also known as tactile memory, haptic involves the very brief memory of a touch. This type of sensory memory lasts for approximately two seconds .
How Does Sensory Memory Work?
During every moment of your existence, your senses are constantly taking in an enormous amount of information about what you see, feel, smell, hear, and taste. While this information is important, there is simply no way to remember each and every detail about what you experience at every moment.
Instead, your sensory memory creates something of a quick "snapshot" of the world around you, allowing you to briefly focus your attention on relevant details.
So just how brief is a sensory memory? Experts suggest that these memories last for three seconds or less .
While fleeting, sensory memory allows us to briefly retain an impression of an environmental stimulus even after the original information source has vanished. By attending to this information, we can then transfer important details into the next stage of memory, which is known as short-term memory .
Sensory memory is an automatic process; it occurs automatically without conscious control:
- Sensory memory is limited in duration and capacity. It lasts very briefly and is almost immediately replaced by new information.
- Researchers have found that attention to the stimulus has only a weak impact on sensory memory.
- The type of memory stored is specific to the sensory modality; in other words, echoic memory stores auditory information, iconic memory stores only visual information, and haptic memory stores only tactile information.
- Information stored in sensory memory includes a great deal of detail.
Examples of iconic memory include situations where you briefly have an image in your head after the sight is gone. For instance, after turning off a flashlight, there is a brief image of the light in your memory. Or, after glimpsing an exit sign on the interstate, you can briefly see the image in your mind.
An example of echoic memory would be the brief memory of the blare of a car horn or the sound of an instrument, even after the noise has ended.
Haptic memory examples might include the brief memory of something brushing up against your skin.
In each instance, the very brief lingering memory you experience allows you to recognize and process the experience.
Sperling's Sensory Memory Experiments
The duration of sensory memory was first investigated during the 1960s by psychologist George Sperling. In a classic experiment, participants stared at a screen and rows of letters were flashed very briefly—for just 1/20th of a second. Then, the screen went blank.
The participants then immediately repeated as many of the letters as they could remember seeing. While most of the participants were only able to report about four or five letters, some insisted that they had seen all the letters but that the information faded too quickly as they reported them.
Inspired by this, Sperling then performed a slightly varied version of the same experiment. Participants were shown the three rows of four letters per row letters for 1/20 th of a second, but immediately after the screen went blank, participants heard either a high-pitched, medium-pitched or low-pitched tone.
If subjects heard the high-pitched tone, they were to report the top row, those who heard the medium-pitched were to report the middle row, and those who heard the low-pitched were to report the bottom row.
Sperling found that participants were able to recall the letters as long as the tone was sounded within one-third of a second of the letter display.
When the interval was extended to over one-third of a second, the accuracy of the letter reports declined significantly, and anything over one second made it virtually impossible to recall the letters.
Sperling suggested that because the participants focused on the indicated row before their visual memory faded, they could recall the information. The recall was nearly impossible when the tone was sounded after sensory memory faded.
Pratte MS. Iconic memories die a sudden death . Psychol Sci . 2018;29(6):877-887. doi:10.1177/0956797617747118
Dubrowski, A. Evidence for haptic memory . World Haptics 2009 - Third Joint EuroHaptics conference and Symposium on Haptic Interfaces for Virtual Environment and Teleoperator Systems, Salt Lake City, UT, USA. doi:10.1109/WHC.2009.4810867
Öğmen H, Herzog MH. A new conceptualization of human visual sensory-memory . Front Psychol . 2016;7:830. doi:10.3389/fpsyg.2016.00830
Tripathy SP, Öǧmen H. Sensory memory is allocated exclusively to the current event-segment . Front Psychol . 2018;9:1435. doi:10.3389/fpsyg.2018.01435
Persuh M, Genzer B, Melara RD. Iconic memory requires attention . Front Hum Neurosci . 2012;6:126. doi:10.3389/fnhum.2012.00126
Sperling G. The information available in brief visual presentations . Psychological Monographs: General and Applied. 1960;74 (11):1-29. doi:10.1037/h0093759
By Kendra Cherry, MSEd Kendra Cherry, MS, is a psychosocial rehabilitation specialist, psychology educator, and author of the "Everything Psychology Book."
Iconic Memory: Definition & Examples
Ayesh Perera
B.A, MTS, Harvard University
Ayesh Perera, a Harvard graduate, has worked as a researcher in psychology and neuroscience under Dr. Kevin Majeres at Harvard Medical School.
Learn about our Editorial Process
Saul McLeod, PhD
Editor-in-Chief for Simply Psychology
BSc (Hons) Psychology, MRes, PhD, University of Manchester
Saul McLeod, PhD., is a qualified psychology teacher with over 18 years of experience in further and higher education. He has been published in peer-reviewed journals, including the Journal of Clinical Psychology.
Olivia Guy-Evans, MSc
Associate Editor for Simply Psychology
BSc (Hons) Psychology, MSc Psychology of Education
Olivia Guy-Evans is a writer and associate editor for Simply Psychology. She has previously worked in healthcare and educational sectors.
On This Page:
Iconic memory is the visual sensory memory register which stores visual images after the extinction of a physical stimulus. While iconic memory contains a huge capacity, it declines rapidly. Information stored in iconic memory generally disappears within half a second (depending on the brightness).
Iconic memory is a sensory memory component involving a fast-decaying visual information store (Sperling, 1960). It provides a coherent yet fleeting representation of our visual perception (Pratte, 2018).
Aristotle was among the earliest individuals to have documented the persistence of a visual representation of an object following its physical extinction (Allen, 1926). He pointed out that experiencing a dream involves afterimages.
In the 18th and 19th centuries, observing the trail of light engendered by a moving stick’s shining ember elicited the curiosity of many researchers. They delved into the empirical analyses of this phenomenon which was subsequently described as visible persistence (Coltheart, 1980).
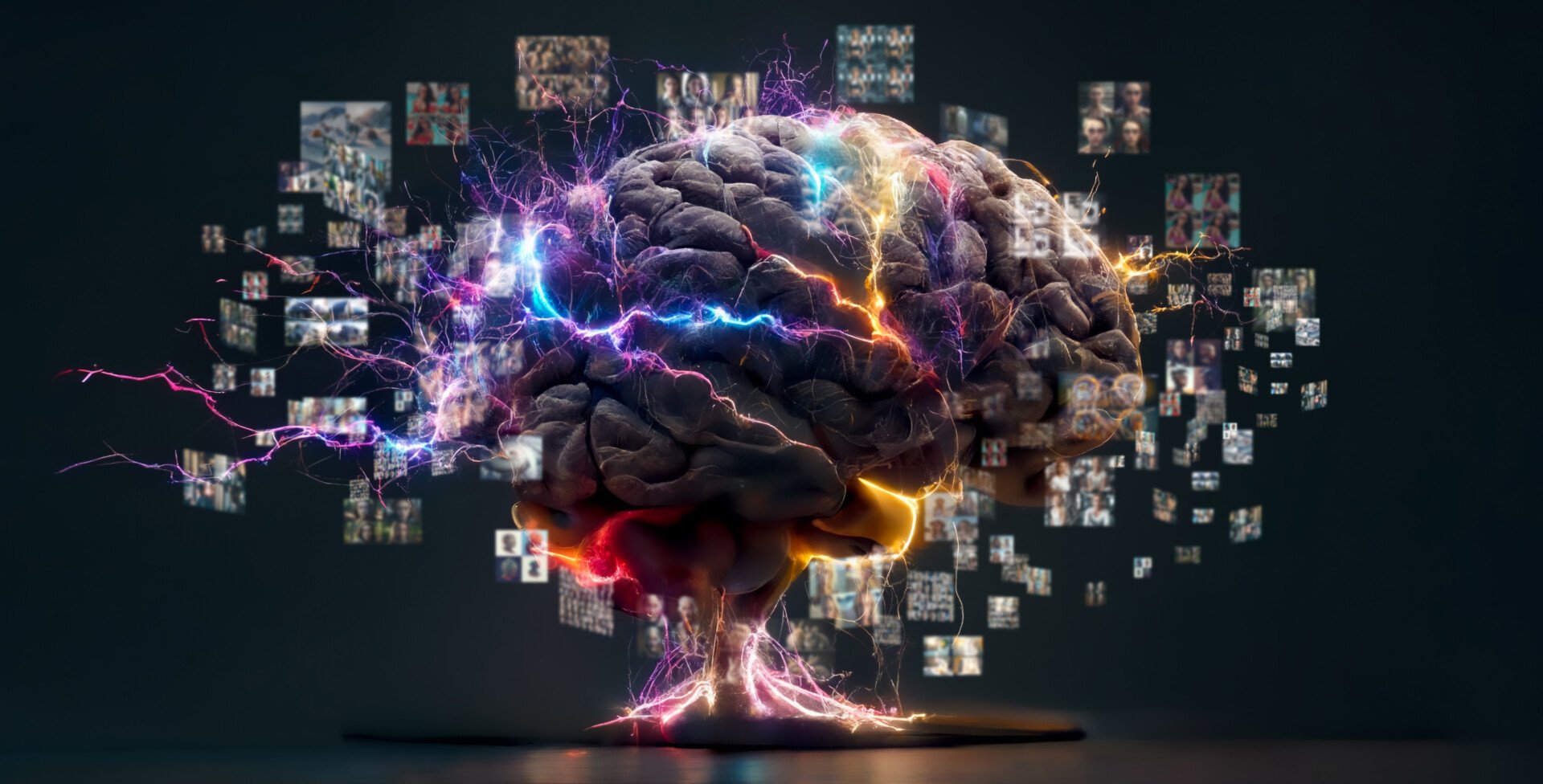
Eventually, in 1960, the American cognitive psychologist George Sperling, via several experiments, confirmed capacity and duration as parts of a memory system called visual sensory memory (Sperling, 1960).
Seven years later, Ulric Neisser introduced the term ‘iconic memory’ to refer to this fast-decaying store of memory (Neisser, 1967).
According to the current understanding of iconic memory, informational persistence and visual persistence embody fundamentally distinct properties, and the former is thought to substantially contribute to visual short-term memory (Coltheart, 1980; Irwin & Yeomans, 1986).
Examples of Iconic Memory
Following are some examples of iconic memory:
- You briefly survey the objects in your bedroom before turning the lights off. The memory of how your surroundings appeared is an example of iconic memory.
- You pass a huge billboard while driving on the highway. Your memory of what you saw on the billboard is an example of iconic memory.
- While riding on the train, you see a lamb grazing in the meadows. The memory of what you briefly beheld is an example of iconic memory.
- You are outside on a dark and rainy night. Suddenly, your surroundings are lit up by a flash of lightning. The fleeting image you saw under the brief glow, which you could subsequently recall, is an example of iconic memory.
Sperling’s Experiments
In his initial experiments conducted in 1960, Sperling presented the observers with a tachistoscopic stimulus comprising various alphanumeric characters for nearly 50 milliseconds (Sperling, 1960).
Afterward, based on a cue, the participants had to recall lines of letters from the display. The performance of memory was compared under whole report and partial report conditions.
What was the difference between Sperling’s full report and partial report tasks?
While the “whole report” required the participants to recall elements according to their original spatial positions, the “partial report” demanded that subsets of the display’s characters be identified based on cued recall at different time intervals. A high, medial, or low tone would indicate which group of characters needed to be reported.
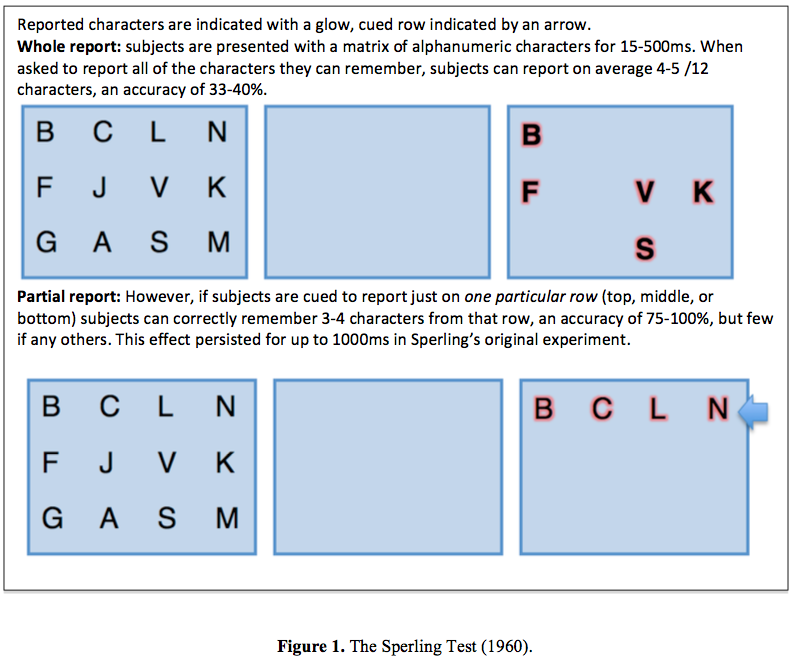
Under the whole report conditions, the participants were generally able to recall about 35% of the characters on display (Sperling, 1960). The findings suggest that the whole report is restricted by a system of memory that has a capacity of 4 to 5 objects.
On the other hand, under the partial report conditions, the participants could recall a specific row in 75% of the trials.
Because the participants were unaware of the row that they would have to recall, the performance herein was tantamount to a random sampling of the memory of the participants for the entire display.
The results herein seemed to suggest that three-fourths of the visual display remained accessible to memory. This outcome signified a manifest increase in iconic memory’s hypothesized capacity.
Iconic Memory and Change Blindness
Change blindness is the phenomenon whereby an alteration introduced to the visual stimulus escapes the notice of the observer (Becker, Pashler & Anstis, 2000). The brief interval between the two successive visual scenes, the latter of which is slightly altered, is called the interstimulus interval.
The length of the interstimulus interval is thought to reduce the iconic memory store. In other words, iconic memory is what enables the detection of changes in a visual scene (Persuh, Genzer & Melara, 2018).
However, lapses in iconic memory, which can be defined as change blindness, are likely to occur in proportion to the length of the interstimulus interval.
Neurology Related to Iconic Memory
The retina’s photoreceptors, the retinal ganglion cells, the middle occipital gyrus, proteins in the brain and various genetic factors impact the functioning of iconic memory.
The visual sensory pathway plays a vital role in iconic memory. The retina’s photoreceptors, namely rods, and cones, remain active beyond a stimulus’ physical offset (Irwin & Thomas, 2008). The transient M-type cells and the sustained P-type cells of the retinal ganglion cells are also involved (Levick & Zacks, 1970).
While the former are active solely during stimulus onset and offset, the latter are active even in between. The primary visual cortex within the occipital lobe, too, plays an important role in the process (Nikolić, Häusler, Singer & Maass, 2009).
Moreover, the middle occipital gyrus shapes iconic memory’s capacity to detect change (Beste, Schneider, Epplen & Arning, 2011). Proteins in the brain and various genetic factors also impact iconic memory’s functioning.
Key Takeaways
- Iconic memory is a sensory memory that stores images for a fraction of a second.
- Iconic memory allows for the retention of visual sensory impressions following the cessation of the original stimulus, with the result that a visual stimulus is subjectively sustained by up to several hundred milliseconds.
- In 1960 George Sperling demonstrated that, for a brief period following the termination of a 50-millisecond display of letters, more information was available than an observer could normally report.
- Future studies have focused on the effects of luminance, duration, contrast, and background level on the clarity and duration of iconic memory storage.
What does iconic memory store?
Iconic memory stores visual information from the environment, allowing for brief and temporary retention of visual stimuli. It is responsible for holding a visual snapshot of the sensory input before further processing and interpretation occur.
What is the duration of iconic memory?
The duration of iconic memory is very brief, typically lasting for a fraction of a second to a few seconds. It is a fleeting and transient form of memory that provides a short-lived representation of visual information before it quickly decays or is overwritten by new incoming stimuli.
Allen, F. (1926). The persistence of vision. American Journal of Physiological Optics, 7, 439–457.
Becker, M. W., Pashler, H., & Anstis, S. M. (2000). The role of iconic memory in change-detection tasks . Perception, 29 (3), 273-286.
Beste, C., Schneider, D., Epplen, J. T., & Arning, L. (2011). The functional BDNF Val66Met polymorphism affects functions of pre-attentive visual sensory memory processes . Neuropharmacology, 60 (2-3), 467-471.
Coltheart, M. (1980). Iconic memory and visible persistence. Perception & psychophysics, 27 (3), 183-228.
Dick, A. O. (1974). Iconic memory and its relation to perceptual processing and other memory mechanisms . Perception & Psychophysics, 16 (3), 575-596.
Irwin, D. E., & Yeomans, J. M. (1986). Sensory registration and informational persistence . Journal of Experimental Psychology: Human Perception and Performance, 12 (3), 343.
Irwin, D. E., & Yeomans, J. M. (1986). Sensory registration and informational persistence. Journal of Experimental Psychology: Human Perception and Performance, 12(3), 343.
Irwin, D., & Thomas, L. (2008). Neural basis of sensory memory. Visual memory , 32-35.
Levick, W. R., & Zacks, J. L. (1970). Responses of cat retinal ganglion cells to brief flashes of light. The Journal of Physiology, 206 (3), 677-700.
Neisser, Ulric (1967). Cognitive Psychology . New York: Appleton-Century-Crofts.
Nikolić, D., Häusler, S., Singer, W., & Maass, W. (2009). Distributed fading memory for stimulus properties in the primary visual cortex. PLoS biology, 7 (12), e1000260.
Persuh, Marjan; Genzer, Boris; Melara, Robert (20 April 2018). Iconic memory requires attention. Frontiers in Human Neuroscience, 6 , 126.
Pratte, M. S. (2018). Iconic memories die a sudden death. Psychological science, 29 (6), 877-887.
Sperling, G. (1960). The information available in brief visual presentations. Psychological monographs: General and applied, 74 (11), 1.
Further Reading
Sperling, G. (1960). The information available in brief visual presentations. Psychological monographs: General and applied, 74(11), 1.
Öğmen, H., & Herzog, M. H. (2016). A new conceptualization of human visual sensory-memory. Frontiers in psychology, 7, 830.
Sligte, I. G., Vandenbroucke, A. R., Scholte, H. S., & Lamme, V. (2010). Detailed sensory memory, sloppy working memory. Frontiers in Psychology, 1, 175.
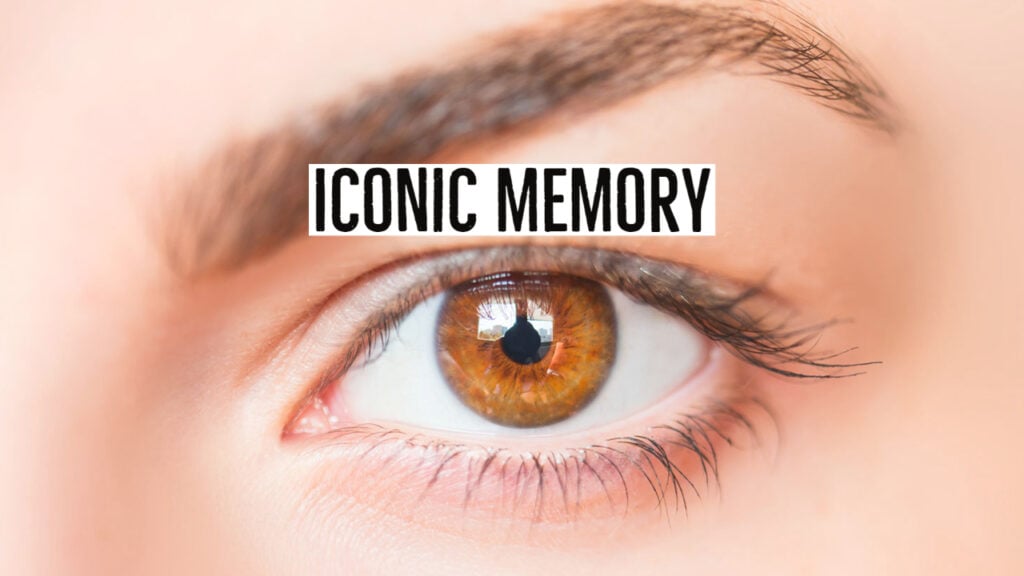
Sensory Memory
- Reference work entry
- pp 3042–3044
- Cite this reference work entry
- Zhong-Lin Lu 2
615 Accesses
Sensory registers
Stimulation of human sense organs is initially represented in sensory memory for a brief period by a literal, labile, and modality-specific neural copy. The term iconic memory stands for the initial representation of visual stimuli, and echoic memory is its counterpart for auditory stimulation (Neisser 1967 ). Sensory memory is often contrasted with short-term memory and working memory which are assumed to be less modality specific, and all of these are distinct from long-term memory. In functional terms, sensory memory is comparable to a register in a computer. In a human, if you want to know what the last sensory input was, you examine the sensory register. The prevailing view of human memory systems is that there are one or more modality-specific sensory registers in each sensory modality, plus a register or registers for short-term and/or working memory, plus functionally distinct long-term memory systems for episodic, semantic, procedural, and...
This is a preview of subscription content, log in via an institution to check access.
Access this chapter
Subscribe and save.
- Get 10 units per month
- Download Article/Chapter or eBook
- 1 Unit = 1 Article or 1 Chapter
- Cancel anytime
- Available as PDF
- Read on any device
- Instant download
- Own it forever
- Available as EPUB and PDF
Tax calculation will be finalised at checkout
Purchases are for personal use only
Institutional subscriptions
Coltheart, M. (1980). Iconic memory and visual persistence. Perception and Psychophysics, 27 (3), 183–228.
Google Scholar
Cowan, N. (1995). Attention and memory: An integrated framework . New York, NY: Oxford University Press.
Crowder, R. G. (1976). Principles of learning and memory . Hillsdale, NJ: Erlbaum.
Gegenfurtner, K. R., & Sperling, G. (1993). Information transfer in iconic memory experiments. Journal of Experimental Psychology: Human Perception & Performance, 19 , 845–866.
Glanzer, M., & Cunitz, A. R. (1966). Two storage mechanisms in free recal. Journal of Verbal Learning and Verbal Behavior, 5 , 351–360.
Long, G. M. (1980). Iconic memory: A review and critique of the study of short-term visual storage. Psychological Bulletin, 88 , 785–820.
Lu, Z. L., Williamson, S. J., & Kaufman, L. (1992a). Human auditory primary and association cortex have differing lifetimes for activation traces. Brain Res, 572 (1–2), 236–241.
Lu, Z. L., Williamson, S. J., & Kaufman, L. (1992b). Behavioral lifetime of human auditory sensory memory predicted by physiological measures. Science, 258 (5088), 1668–1670.
Massaro, D. W. (1975). Experimental psychology and information processing . Chicago, IL: Rand McNally.
Massaro, D. W., & Loftus, G. R. (1996). Sensory and perceptual storage: data and theory. In E L Ba R A Bjork (Ed.), Memory . San Diego, CA: Academic Press.
Neisser, U. (1967). Cognitive psychology . East Norwalk: Appleton-Century-Crofts.
Sperling, G. (1960). The information available in brief visual presentation. Psychological Monographs, 74 , 1–29.
Treisman, A. (1964). Monitoring and storage of irrelevant messages in selective attention. Journal of Verbal Learning and Verbal Behavior, 3 , 449–459.
Uusitalo, M. A., Williamson, S. J., & Seppa, M. T. (1996). Dynamical organisation of the human visual system revealed by lifetimes of activation traces. Neuroscience Letters, 213 , 149–152.
Weinberger, N. M., Ashe, J. H., Metherate, R., McKenna, T. M., Diamond, D. M., & Bakin, J. (1990). Retuning auditory cortex by learning: a preliminary model of receptive field plasticity. Concepts in Neuroscience, 1 , 91–132.
Wickelgren, W. A. (1969). Associative strength theory of recognition memory for pitch. Journal of Mathematical Psychology, 6 , 13–61.
Download references
Author information
Authors and affiliations.
Departments of Psychology, University of Southern California, 3620 South McClintock Av., Room 501, 90089–1061, Los Angeles, CA, USA
Zhong-Lin Lu
You can also search for this author in PubMed Google Scholar
Corresponding author
Correspondence to Zhong-Lin Lu .
Editor information
Editors and affiliations.
Faculty of Economics and Behavioral Sciences, Department of Education, University of Freiburg, 79085, Freiburg, Germany
Norbert M. Seel
Rights and permissions
Reprints and permissions
Copyright information
© 2012 Springer Science+Business Media, LLC
About this entry
Cite this entry.
Lu, ZL. (2012). Sensory Memory. In: Seel, N.M. (eds) Encyclopedia of the Sciences of Learning. Springer, Boston, MA. https://doi.org/10.1007/978-1-4419-1428-6_257
Download citation
DOI : https://doi.org/10.1007/978-1-4419-1428-6_257
Publisher Name : Springer, Boston, MA
Print ISBN : 978-1-4419-1427-9
Online ISBN : 978-1-4419-1428-6
eBook Packages : Humanities, Social Sciences and Law Reference Module Humanities and Social Sciences Reference Module Education
Share this entry
Anyone you share the following link with will be able to read this content:
Sorry, a shareable link is not currently available for this article.
Provided by the Springer Nature SharedIt content-sharing initiative
- Publish with us
Policies and ethics
- Find a journal
- Track your research
An official website of the United States government
Official websites use .gov A .gov website belongs to an official government organization in the United States.
Secure .gov websites use HTTPS A lock ( Lock Locked padlock icon ) or https:// means you've safely connected to the .gov website. Share sensitive information only on official, secure websites.
- Publications
- Account settings
- Advanced Search
- Journal List
A New Conceptualization of Human Visual Sensory-Memory
Haluk öğmen, michael h herzog.
- Author information
- Article notes
- Copyright and License information
Edited by: Britt Anderson, University of Waterloo, Canada
Reviewed by: Ilja G. Sligte, University of Amsterdam, Netherlands; Jan Brascamp, Michigan State University, USA
*Correspondence: Haluk Öğ[email protected]
This article was submitted to Perception Science, a section of the journal Frontiers in Psychology
Received 2016 Feb 2; Accepted 2016 May 18; Collection date 2016.
This is an open-access article distributed under the terms of the Creative Commons Attribution License (CC BY). The use, distribution or reproduction in other forums is permitted, provided the original author(s) or licensor are credited and that the original publication in this journal is cited, in accordance with accepted academic practice. No use, distribution or reproduction is permitted which does not comply with these terms.
Memory is an essential component of cognition and disorders of memory have significant individual and societal costs. The Atkinson–Shiffrin “modal model” forms the foundation of our understanding of human memory. It consists of three stores: Sensory Memory (SM), whose visual component is called iconic memory, Short-Term Memory (STM; also called working memory, WM), and Long-Term Memory (LTM). Since its inception, shortcomings of all three components of the modal model have been identified. While the theories of STM and LTM underwent significant modifications to address these shortcomings, models of the iconic memory remained largely unchanged: A high capacity but rapidly decaying store whose contents are encoded in retinotopic coordinates, i.e., according to how the stimulus is projected on the retina. The fundamental shortcoming of iconic memory models is that, because contents are encoded in retinotopic coordinates, the iconic memory cannot hold any useful information under normal viewing conditions when objects or the subject are in motion. Hence, half-century after its formulation, it remains an unresolved problem whether and how the first stage of the modal model serves any useful function and how subsequent stages of the modal model receive inputs from the environment. Here, we propose a new conceptualization of human visual sensory memory by introducing an additional component whose reference-frame consists of motion-grouping based coordinates rather than retinotopic coordinates. We review data supporting this new model and discuss how it offers solutions to the paradoxes of the traditional model of sensory memory.
Keywords: sensory memory, iconic memory, modal model, non-retinotopic memory, non-retinotopic processes

Introduction
Modal model of human memory.
The realization that human memory is not a unitary process but consists of multiple stores with distinct characteristics led to the Atkinson–Shiffrin, or the “modal” model of human memory (Atkinson and Shiffrin, 1968 ). As shown in Figure 1 , this model consists of three major stores: The input is first stored in sensory memory (SM), which exhibits a very large capacity, but can maintain information only for a few hundred milliseconds. A subset of the contents of this rapidly decaying memory is transferred to Short-Term Memory (STM; also known as Working Memory WM). STM is severely limited in capacity and can hold information for several seconds to minutes. Finally, information is stored in Long-Term Memory (LTM), a store with very large capacity, capable of holding information as long as one's lifetime. Since its inception, the STM and LTM components of the modal model have undergone significant modifications (review: Baddeley, 2007 ), while SM has remained largely unchanged 1 .
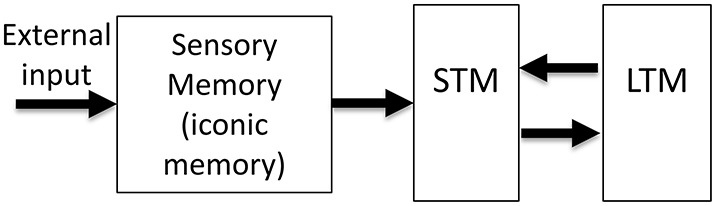
The Atkinson-Shiffrin, or the “modal model” of human memory . The external input is first stored in a large capacity but rapidly decaying store, called Sensory Memory (SM). Alternative terms for this stage include sensory register and sensory store. The visual component of SM is also called iconic memory. A distinction has been made between visible persistence vs. informational persistence in visual SM. Some authors use the term iconic memory only for the informational persistence component of visual SM, whereas others use for both visual and informational components. A subset of the contents of SM is transferred into a more durable (few seconds) but severely capacity-limited store, called Short-Term Memory (STM). Alternative terms for this stage include Short-Term Store, or Working Memory (WM). Some authors make a distinction between the use of the terms STM and WM. Finally, the contents of STM are transferred to Long-Term Memory (LTM), also called Long-Term Store, a store with very long duration and very large capacity.
Sensory (iconic) memory
The SM component of the modal model is based on Sperling's work in 1960s (Sperling, 1960 ; Averbach and Sperling, 1961 ). By using the partial-report technique, Sperling showed that a large-capacity visual memory stores information for few hundred milliseconds (Sperling, 1960 ) and more recent studies indicate that this information is not implicit and unconscious but rather directly reflects the phenomenal richness of our visual experience (Vandenbroucke et al., 2014 ). Early information processing theories viewed SM as a real-time buffer, which briefly stores the inputs impinging on the retina to allow attentional mechanisms to select a subset of this information for transfer to the limited capacity WM. However, subsequent analyses taking into account the properties of dynamic ecological viewing conditions showed that SM cannot fulfill this function during normal viewing conditions when objects or the subject are in motion; in fact, SM appears to be a hindrance to vision (Haber, 1983 ). A fundamental characteristic of iconic memory is that its contents are encoded in retinotopic coordinates (Haber, 1983 ; Irwin et al., 1983 , 1988 ; Jonides et al., 1983 ; Rayner and Pollatsek, 1983 ; van der Heijden et al., 1986 ; Sun and Irwin, 1987 ). While a retinotopically encoded memory can serve a useful function when the observer and the objects in the environment are all static, it cannot store any meaningful information when the observer's eyes, head, body and external objects are in motion. Any relative motion between the observer's retinae and the external environment will cause a shift in retinotopic coordinates for the stimulus received by SM. These shifts, in turn, will cause blurring and inappropriate integration of information over space and time: A briefly presented stimulus remains visible for about 120 ms after its offset under normal viewing conditions (Coltheart, 1980 ), a phenomenon known as visible persistence (the visible component of SM 2 ). Hence, if the input shifts in retinotopic coordinates, it will create partially processed copies of the stimulus that will be superimposed upon each other at different retinotopic locations, creating a blurred version of the stimulus. For example, given a visible persistence duration of 120 ms, an object moving at 8.3°/s will generate a blur trail of 1°. This motion blur is similar to pictures of moving objects taken by a camera at relatively slow shutter speeds mimicking the duration of visible persistence (Figure 2 ). Similarly, when the observer moves her head, body, and eyes, the retinotopic shift of stimuli engenders multiple blurred copies superimposed upon each other in SM. Since movements of the subject and the objects are characteristics of ecological normal viewing conditions, the emerging consensus has been that a retinotopically encoded memory cannot serve any useful function under normal viewing conditions. To explain our relatively sharp and clear percepts under normal viewing conditions, there have been several attempts to identify a spatiotopic version of this memory (Davidson et al., 1973 ; Ritter, 1976 ; White, 1976 ; Wolfe et al., 1978a , b ; Breitmeyer et al., 1982 ; Jonides et al., 1982 ; McRae et al., 1987 ); however, these were unsuccessful (Haber, 1983 ; Irwin et al., 1983 , 1988 ; Jonides et al., 1983 ; Rayner and Pollatsek, 1983 ; van der Heijden et al., 1986 ; Sun and Irwin, 1987 ) and this area of research has been stagnant for half-century.
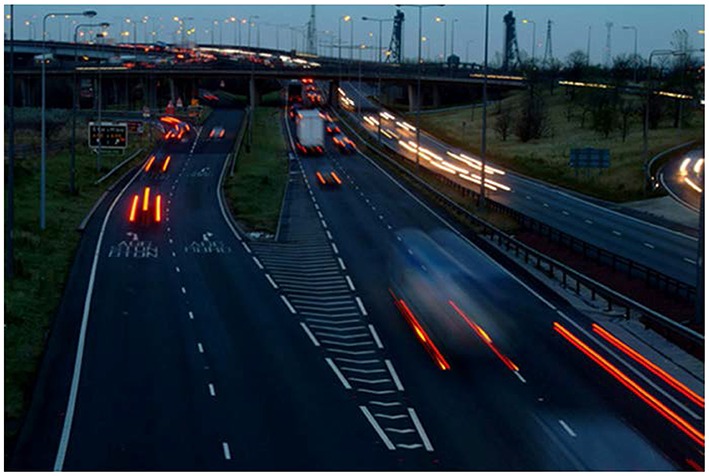
A picture illustrating deleterious effects of a retinotopic Sensory Memory (rSM) . As an object moves in the retinotopic space, its trace in rSM will create blur, as is the case for moving objects in the scene. Retinotopically shifted copies of the stimulus are superimposed and integrated, creating formless, ghost—like, appearances for moving objects. Similarly, the movements of observer's eyes, head, and body will induce retinotopic shifts in the image, blurring the whole scene and making the scene unrecognizable with a ghost-like appearance. Original photo from FreeFoto.com by permission.
To move forward, three fundamental questions need to be addressed:
Q1. How does the visual system process and store information non-retinotopically over space and time?
Q2. How does the visual system control deleterious effects of retinotopic sensory memory?
Q3. What purpose does a retinotopic sensory memory serve?
In the following we provide answers to these questions. First, to put retinotopy in the context of visual perception, in Section Metacontrast and Anorthoscopic Perception: A Retinotopically Extended Representation is Neither Sufficient nor Necessary for Vision we present evidence showing that retinotopic representations are neither sufficient nor necessary for visual perception. In Sections Sequential Metacontrast: Non-retinotopic Information Storage and Processing and The Ternus-Pikler Paradigm to Probe Retinotopic and Non-retinotopic Processes, we review briefly two experimental paradigms that we have used to demonstrate the existence of a non-retinotopic memory. Based on these findings, in Section A New Conceptualization of Human Sensory Memory, we present a modified version of the modal model with a new sensory memory component. In Section Paradoxes of Retinotopic Sensory Memory Revisited, we revisit the paradoxes associated with the retinotopic sensory memory and discuss how the new model offers resolutions to these paradoxes.
Non-retinotopic information processing and storage
Metacontrast and anorthoscopic perception: a retinotopically extended representation is neither sufficient nor necessary for vision.
Assume that one briefly flashes a supra-threshold stimulus; the observer will clearly perceive the shape of this stimulus. Assume now that a second stimulus is flashed after this stimulus in a way that it surrounds but does not spatially overlap with the first stimulus. This second stimulus can render the first one completely invisible. This phenomenon is known as visual masking, which refers to the reduced visibility of one stimulus (target), due to the presence of another stimulus (mask) (Bachmann, 1994 ; Breitmeyer and Öğmen, 2000 , 2006 ). Metacontrast is a specific type of visual masking, in which the target and mask do not overlap spatially. Hence in metacontrast, the target maintains its retinotopic representation, i.e., the mask does not reduce the visibility of the target by directly occluding the retinotopic representation of the target. Hence a retinotopic representation of a stimulus is not sufficient for its perception or storage. The mask may be interfering indirectly with the retinotopic representation of the mask; but why would the visual system suppress a perfectly visible stimulus when it is embedded in a dynamic context? In the following sections, we will re-visit visual masking and its role in controlling sensory memory in Sections Sequential Metacontrast: Non-retinotopic Information Storage and Processing and How the Visual System Controls Deleterious Effects of rSM: Motion Deblurring to answer the questions Q1 and Q2 above.
Anorthoscopic perception, or slit viewing, is an experimental paradigm that derives its name from the anorthoscope, a device invented by Plateau in the nineteenth century (Plateau, 1836 ). Since its invention, variants of this device have been used to study human perception (e.g., Zöllner, 1862 ; Parks, 1965 ; Rock, 1981 ; Morgan et al., 1982 ; Sohmiya and Sohmiya, 1994 ; Öğmen, 2007 ; Aydin et al., 2008 , 2009 ; Agaoglu et al., 2012 ). As depicted in Figure 3 , a moving stimulus is viewed behind a narrow slit. All spatial information about the moving stimulus is restricted into a very narrow retinotopic strip. At any given time, only a very narrow spatial structure of the stimulus is visible. In other words, there is no spatially extended retinotopic representation for the moving stimulus. Moreover, as the stimulus moves, different parts of the object's shape fall onto the same retinotopic area. Hence, the contents of a retinotopic SM will consist of all stimulus parts mixed and blended into each other within the slit area. Yet, observers are able to spatiotemporally integrate the slit views to construct the spatially extended form of the moving stimulus in the absence of a retinotopically extended representation of the stimulus. Hence, anorthoscopic perception shows that a spatially extended retinotopic representation is not necessary for the perception of spatial form. Moreover, it also shows that, since information about different parts of the shape are shown at different time instants, the visual system is able to store this information and integrate it non-retinotopically in order to build the complete spatial layout of the stimulus. This indicates some type of non-retinotopic memory (Haber, 1983 ) but until recently it was not clear how it may be operating and its relation to the more traditional retinotopic SM. We will revisit anorthoscopic perception in Section Anorthoscopic Perception.
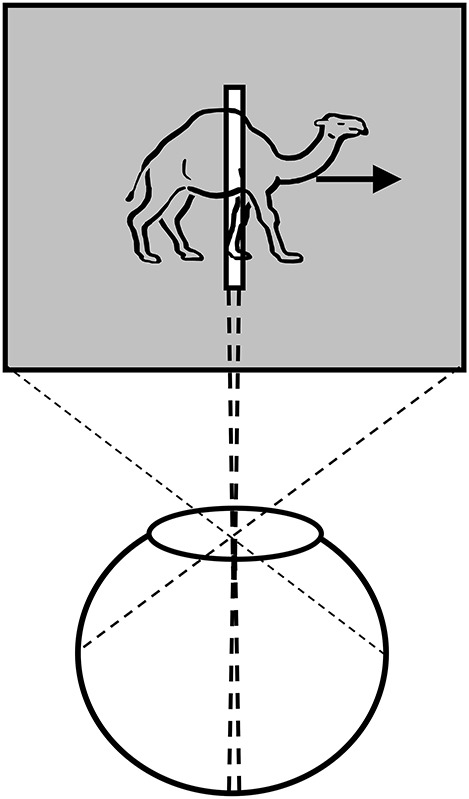
Anorthoscopic perception . A stimulus is moved behind an opaque region with only a very narrow opening in the form of a slit. As the object moves behind the slit, samples of its form taken along the slit fall unto the same narrow retinotopic region. Hence, these different narrow two-dimensional samples will be superimposed and integrated in the retinotopic sensory memory, making the perception of spatially extended form impossible. From Öğmen ( 2007 ).
Sequential metacontrast: non-retinotopic information storage and processing
Sequential metacontrast (Piéron, 1935 ; Otto et al., 2006 ) is a special case of metacontrast, consisting of multiple target and mask pairs as shown at the bottom of Figure 4 . A central target is presented first, followed by two spatially flanking masks, which in turn are followed by lateral masks on one side, etc. Observers perceive two motion streams originating from the center, one to the left, and one to the right. With the appropriate choice of stimulus parameters, the central element can be completely masked making observers unable to tell whether it is presented or not (Otto et al., 2006 ). In order to test non-retinotopic storage and integration of information, we introduced a feature into this invisible central element in the form of a vernier offset (called “probe-vernier”), with a random spatial shift between its two segments, left or right, from trial to trial. Observers were asked to report the perceived vernier-offset in the left motion stream. Observers did not know if and where vernier offset(s) were presented in the display. In Figure 4A , all flanking lines are non- offset and the responses of the observers are above chance level with the offset of the probe-vernier. This indicates that the central invisible probe-vernier's offset direction is stored in a non-retinotopic memory and attributed to the left motion stream, a process that we call feature attribution. In Figure 4B , a vernier of opposite offset-direction is introduced into the left stream (in reference to the probe-vernier, this is called “anti-vernier” hereafter, because its offset direction is always opposite to that of the probe-vernier). Now, the agreement of observer's response with the offset direction of the probe-vernier is near 50%, i.e., the point of equal strength. This indicates that the two verniers are integrated in the non-retinotopic memory and as a result they cancel each other. Finally, in Figure 4C we show that this integration is specific to the motion stream, i.e., the two verniers are integrated only if they belong to the same motion stream . The probe-vernier is symmetric with respect to leftward and rightward motion streams; hence it will be attributed to both streams. The anti-vernier will be integrated with the probe vernier only in the specific motion stream where it appears. In Figures 4B,C , it will be integrated exclusively within the leftward and rightward motion streams, respectively. Since the observer is reporting the leftward motion stream, the integration is revealed in observer's response in Figure 4B but not in Figure 4C . Taken together, these results show that information presented at the central retinotopic location is stored in memory and is integrated with the information presented at other retinotopic locations according to the motion of stimuli, hence, providing evidence for a non-retinotopic memory that depends on stimulus motion. Additional results supporting this finding (with multiple vernier's inserted at multiple locations) can be found in Otto et al. ( 2006 , 2009 , 2010a , b ).
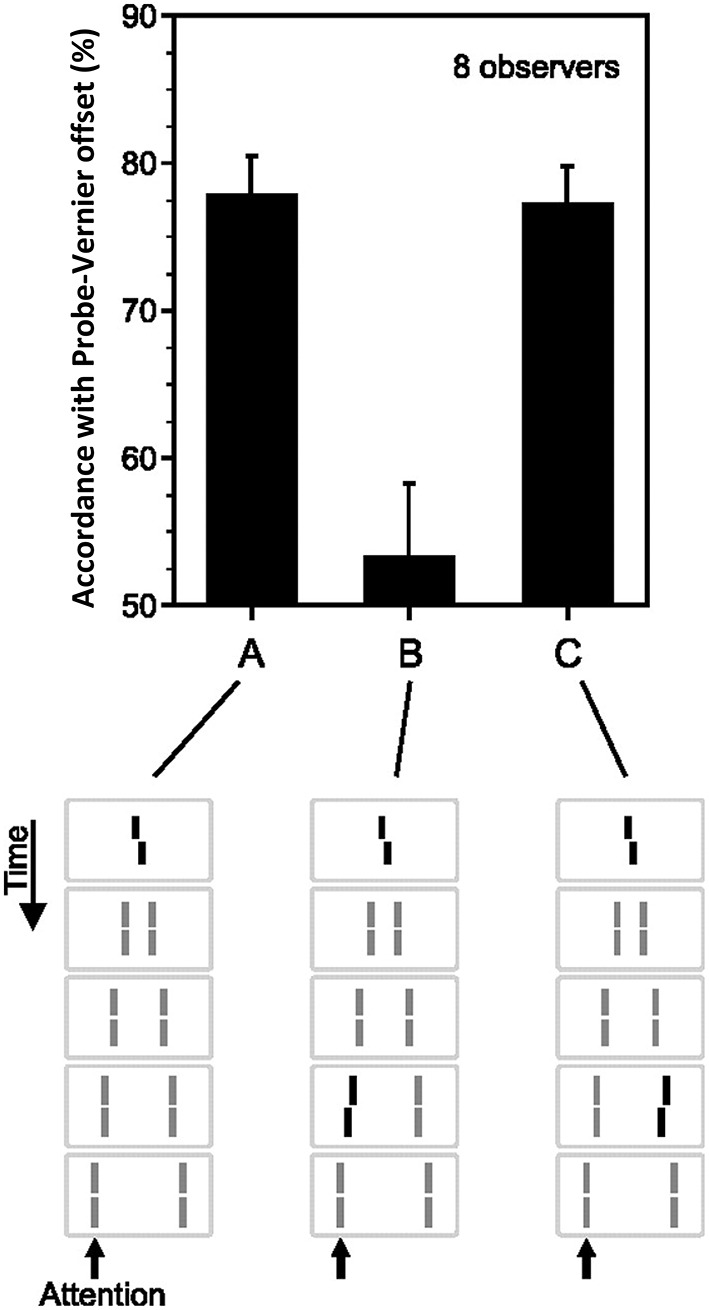
Non-retinotopic memory revealed by sequential metacontrast . The stimulus, shown at the bottom of the figure, consists of a central vernier, which is followed in time by a succession of spatially adjacent verniers. Observers perceive two motion streams, one to the left and one to the right, emerging from the center. A vernier offset, called the “probe-vernier,” is introduced to the central element. Observers report the perceived vernier offset (left or right) in the left motion stream (indicated by the arrow). In (A) , none of the flanking verniers has an offset. Observers' responses are in agreement with the offset of the probe-vernier in about 80% of the trials. In (B) , a vernier of opposite offset with respect to the probe vernier, called “anti-vernier,” is introduced to the left motion stream. Now, the agreement of the observers' response with the probe-vernier is near chance. This indicates that the information about the probe-vernier is stored in memory and is integrated with the anti-vernier so that the two cancel each other. The important point is that the storage and integration is non-retinotopic, since the probe-vernier and anti-vernier are presented at different retinotopic locations. In (C) , the anti-vernier is inserted to the rightward motion stream. In this case, the probe-vernier and anti-vernier are not integrated, showing that non-retinotopic storage and integration is specific to the motion stream. Adapted from Otto et al. ( 2006 ); the Association for Research in Vision and Ophthalmology (© ARVO).
A methodological difference between traditional studies of memory and the sequential metacontrast paradigm outlined above is the ways cues are used. In the partial-report technique, after the offset of the stimulus, a cue is delivered (with a delay) to indicate which item(s) to report (Sperling, 1960 ). As soon as the cue is delivered, the observer can initiate the reporting process. More recent studies combined change-detection paradigms with retro -cueing to investigate memory processes, in particular STM (e.g., Griffin and Nobre, 2003 ; Sligte et al., 2008 ; Hollingworth and Maxcey-Richard, 2013 ; Rerko et al., 2014 ; van Moorselaar et al., 2015 ). In these studies, an array of items is presented, followed by a retro-cue, and finally by a comparison item or display. The task of the observer is to report whether the cued item has changed. Hence, in this paradigm, the cue itself is not sufficient to initiate the response. Sperling's original purpose for introducing the cue was to design a partial report task so as to avoid the decay of information during the time it takes to report the contents of the memory. In addition to indexing the items to be reported, cues also guide attention and hence allow the examination of the role attention may play in the storage, maintenance, or transfer of information in memory. For example, a retro-cue indicates to the observer which particular memory item to attend in order to complete the impending comparison.
We have combined cueing with sequential metacontrast to examine the role of attention in non-retinotopic memory (Otto et al., 2010a ). In a first experiment, we used a stimulus as shown in Figure 4 . The stimulus could contain only a central vernier (as in Figure 4A ), a central and a flanking vernier (as in Figures 4B,C ), or only a flanking vernier. In the experiment described in Figure 4 , the observers were instructed at the beginning of the block of trials which motion stream to attend (Otto et al., 2006 ). In Otto et al. ( 2010a ), we used an auditory cue that indicated which motion stream (leftward or rightward) to attend. We varied the timing of the auditory cue with respect to the stimulus. When the cue was delivered before stimulus onset, observers focused their attention exclusively on the cued stream. By delaying the cue, we could control when unifocal attention could be devoted to a particular motion stream. Accordingly, the cue could focus attention preferentially on the central or the flanking vernier depending on its timing with respect to the onset of the central and flanking vernier. Our results showed that neither the timing nor the distribution of attention (focused on one stream vs. distributed to both streams) had any specific effect on non-retinotopic feature integration. These findings indicate that attention cannot directly access single lines and mandatory feature integration occurs within the attended motion stream.
The ternus-pikler paradigm to probe retinotopic and non-retinotopic processes
Whereas the sequential metacontrast paradigm provides evidence for non-retinotopic memory, it does not pit directly retinotopic and non-retinotopic processes against each other. In order to achieve this goal, we developed an experimental paradigm that can pit directly retinotopic and non-retinotopic memories against each other, while revealing the direct role of motion in the process. To this end, we modified a stimulus paradigm developed by Gestalt psychologists Ternus and Pikler (Pikler, 1917 ; Ternus, 1926 ). Figure 5A shows a basic Ternus-Pikler display. The first frame contains three elements. After an inter- stimulus interval (ISI), these three elements are shifted to the right by one inter-element distance so that two of the elements overlap retinotopically across the two frames (these retinotopically overlapping elements allow the testing of retinotopic information storage and integration). For small values of ISI, observers report seeing the leftmost element of the first frame move to the rightmost element of the second frame, while the other two elements appear stationary (Figure 5B ). This percept is called “element motion” (Pantle and Picciano, 1976 ). For longer ISIs, observers report seeing all three elements moving in tandem to the right as a group (Figure 5C ). This percept is called “group motion.” These motion-based non-retinotopic correspondences between the elements in the two frames allow the testing of motion-based, non-retinotopic information storage and integration. The probe-vernier was inserted to the central element of the first frame as shown in Figure 5D -left (Öğmen et al., 2006 ). We asked observers to report the perceived offset-direction for elements in the second frame, numbered 1, 2, and 3 in Figure 5D -left. None of these elements contained a vernier offset and naïve observers did not know where the probe-vernier was located. Consider first the control condition in Figure 5E , obtained by removing the flanking elements from the two frames. In this case no motion is perceived. Based on the traditional retinotopic iconic memory account, the information about the probe vernier should be stored at its retinotopic location and it should be integrated with element 1 in the second frame, which occupies the same retinotopic location. Thus, the agreement of observers' responses with the direction of probe-vernier offset should be high for element 1 and low for element 2. In agreement with the large body of findings on iconic memory, this is indeed what we found (data in Figure 5E -right). A retinotopic iconic memory predicts the same outcome for the Ternus-Pikler display regardless of whether element or group motion is perceived, as long as the ISI is within the time-scale of iconic memory. On the other hand, if there is a memory mechanism that stores and integrates information non-retinotopically according to motion grouping relations (Figures 5B,C ), one would expect the probe vernier to integrate with element 1 in the case of element motion (Figure 5B ) and with element 2 in the case of group motion (Figure 5C ). Our results supported the predictions of the non-retinotopic motion- based memory hypothesis ( 5D -right). Additional results supporting this finding (with multiple vernier's inserted at multiple locations) can be found in Öğmen et al. ( 2006 ), Scharnowski et al. ( 2007 ), Otto et al. ( 2008 ), Boi et al. ( 2009 , 2011 ), and Noory et al. ( 2015a , b ).
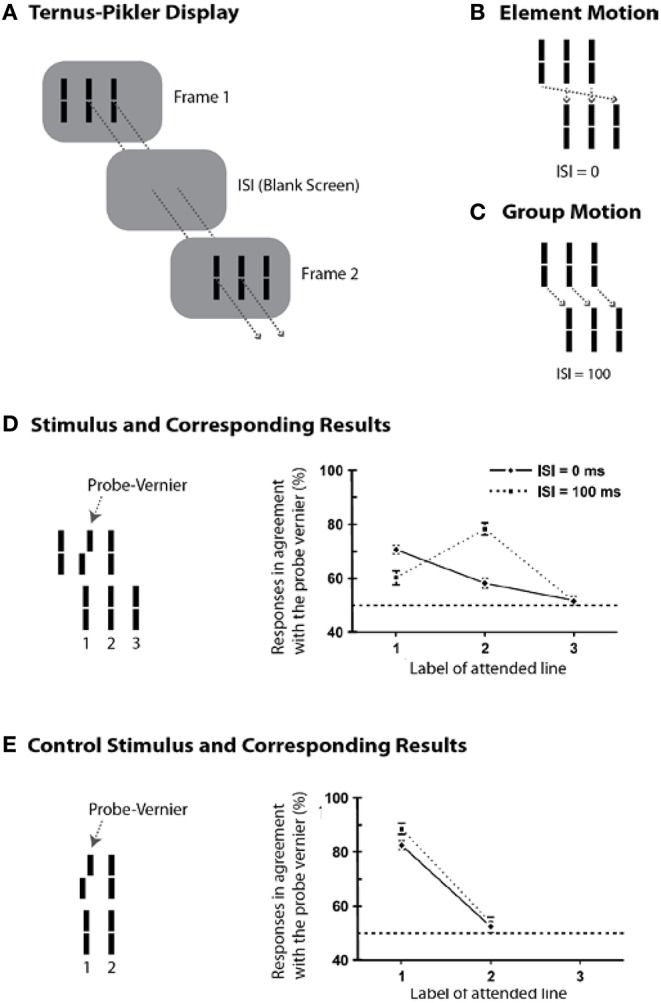
The Ternus-Pikler paradigm to pit directly retinotopic processes against non-retinotopic processes. (A) The Ternus-Pikler display consists of three elements shown in a first frame, followed by a blank screen, and finally another frame where the three elements are shifted by one inter-element distance so that two of the elements in the two stimulus frames fall exactly on the same retinotopic locations. When the duration of the blank screen, i.e., the Inter-Stimulus Interval (ISI) between the two stimulus frames is short, “element motion” is perceived: As depicted by the arrows in (B) , the retinotopically overlapping elements are perceived stationary whereas the leftmost element in the first frame is perceived to move to the rightmost element in the last frame. When ISI is long, “group motion” is perceived: As depicted by the arrows in (C) , the three elements are perceived to move as a group. (D) A probe-vernier is inserted to the central element in the first frame and observers reported the perceived vernier offset for elements marked 1, 2, and 3 in the second frame. In the retinotopic memory, the probe-vernier should be integrated to element 2, which shares the same retinotopic location, regardless of the value of ISI as long as it is within the integration time-window of SM. However, if memory is non-retinotopic and is based on a reference-frame that follows motion grouping, then the probe-vernier should be integrated to element 1 in the case of element motion and to element 2 in the case of group motion (see the motion correspondences in B , C ). The results, shown on the right, support the predictions of motion-grouping based non-retinotopic memory. (E) In the control display, the flanking elements are removed and no motion is perceived. In this case, both retinotopic and non-retinotopic memories predict the same outcome, namely, the probe-vernier should be integrated to element 1 for both ISI values. This is indeed what the results show. Adapted by permission from Öğmen et al. ( 2006 ).
A new conceptualization of human sensory memory
Extensive research supports the existence of a retinotopic sensory memory (review: Coltheart, 1980 ). The research reviewed in the previous section supports the existence of a non-retinotopic, motion-based, sensory memory. Taken into account these recent findings, we modified the sensory memory component of the modal model by introducing a non-retinotopic store (Figure 6 ). To be consistent with the terminology used in the literature, we keep the term iconic memory for the retinotopic component of sensory memory and also refer to this component as the “ retinotopic Sensory Memory” ( r SM). We call the newly introduced non-retinotopic component, the “ non-retinotopic Sensory Memory” ( nr SM).
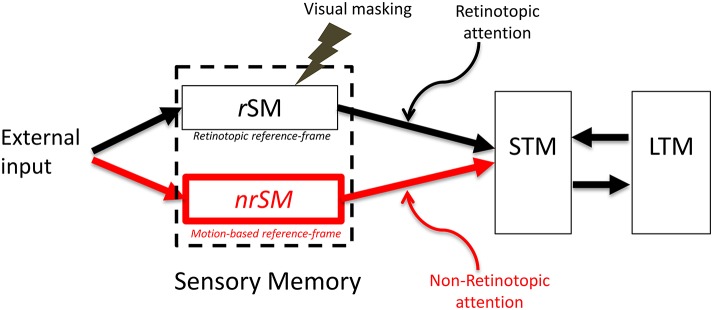
The proposed new model . The SM stage of the modal model is modified to include two stores, a retinotopic SM ( r SM), corresponding to the SM in the modal model and an additional non-retinotopic SM ( nr SM). The reference-frame for r SM is anchored in retinotopic coordinates, whereas the reference-frame of nr SM is non-retinotopic, flexible and based on motion groupings in the stimulus. Hence, nr SM can have simultaneously multiple reference-frames depending on how the stimulus generates motion groupings (see Figure 7 , for an example). The contents of r SM can be suppressed by visual masking whereas nr SM is immune to masking. Information from r SM and nr SM is transferred to STM through retinotopic and non-retinotopic attention mechanisms.
Below, we discuss the fundamental properties of the new model and the key differences between its r SM and nr SM components. Specifically, we will discuss the differences in terms of reference-frames used in each, how masking affects the contents of each memory component, the distinct but complementary roles masking and motion play with respect to these two components, and finally the influence of attention mechanisms:
Retinotopic vs. motion-based reference-frames : Whereas the reference-frame, or the coordinate system, of rSM is anchored in retinotopic coordinates, nrSM uses motion-grouping-based non-retinotopic reference-frames or coordinate systems. Figure 7 depicts the operations underlying nrSM. At a first stage, motion is analyzed within retinotopic representations and motion vectors are grouped according to Gestalt grouping principles (e.g., common fate). For example, in Figure 7 , the moving dots are grouped into two distinct groups based on their direction of motion. For each group, a common motion vector is computed and this common motion vector serves as the reference-frame according to which the contents of memory are encoded. As illustrated in the example, multiple motion groupings can be extracted simultaneously across the visual field and hence nrSM can contain multiple reference-frames, unlike rSM which has a single reference-frame anchored in retinotopic coordinates.
Immunity to masking: In the experiments discussed in Section Sequential Metacontrast: Non-retinotopic Information Storage and Processing, the probe-vernier can be completely masked; however, the information about its vernier-offset is not masked since it is integrated to other verniers in the motion stream and observers can reliably report the direction of vernier offset in behavioral experiments. By using the Ternus–Pikler display, we tested directly whether masking operates in retinotopic coordinates and whether nrSM is susceptible to masking (Noory et al., 2015b ). Our results showed that masking operates in retinotopic coordinates and nrSM is immune to masking (Noory et al., 2015b ). Hence, unlike rSM whose contents are suppressed by masking (Averbach and Coriell, 1961 ; Averbach and Sperling, 1961 ; Coltheart, 1980 ), the contents of nrSM are immune to masking Noory et al., 2015b
Distinct and complementary roles of masking and motion in sensory memory : Masking “turns off” rSM whereas motion “activates” nrSM 3 . To test the proposed distinct but complementary roles of masking and motion, we determined the correlations between the non-retinotopic storage and integration in nrSM (we call this effect “feature attribution” since features are not perceived according to their retinotopic coordinates but are attributed non-retinotopically to motion streams), masking, and motion (Breitmeyer et al., 2008 ). The first frame contained a vernier pair presented to the left of the fixation cross. The second frame contained a vernier pair presented to the right of the fixation cross. Offsets were introduced so that features (vernier offset) either changed or remained the same across the two frames (see Figure 8 ). In the feature-attribution task, subjects judged the vernier pair presented in the second frame and reported whether the upper and lower verniers in the second frame were the same or different (examples highlighted by dashed ovals). For example, the correct response is “same” for the rightmost stimulus sequence in the top panel of Figure 8 and “different” for the rightmost stimulus sequence in the bottom panel. On trials in which feature attribution occurred, a larger number of misidentifications of the vernier pair presented in the second frame would be expected when the stimulus sequences had feature changes than when they did not. Hence, the difference between the numbers of misidentifications in the no-change and feature-change conditions provide an index of feature attribution, with larger differences corresponding to stronger feature attribution. Since feature attribution requires temporal storage and non-retinotopic integration, it measures rSM. In the backward-masking task, subjects judged the vernier pair in the first frame and reported whether the upper and lower verniers were the same or different (examples highlighted by dotted ovals). In the apparent motion task, observers rated the strength of smooth apparent motion, using a scale ranging from 0 (no motion) to 5 (optimal smooth motion). Figure 8B shows the correlations between these three variables computed across several values of stimulus-onset asynchronies between the two frames. As one can see from the figure, feature attribution correlated strongly with motion (significance: p < 0.01 for bivariate correlation and p < 0.03 for partial correlation) while the correlation of feature attribution with masking was weaker and not significant ( p > 0.175 for bivariate correlation and p < 0.295 for partial correlation). Thus, these results support that the operation of nr SM has strong correlation with motion, which according to our theory constitutes its reference-frame, whereas the effect of masking is related to the operation of r SM.
Retinotopic vs. non-retinotopic attention mechanisms: Attention is a key process that controls the transfer of information from SM to STM and various lines of evidence suggest that temporal dynamics of SM plays a fundamental role in determining how attention can select information from SM for transferring into STM (Wutz and Melcher, 2014 ). Attentional processes can be classified into two broad types, endogenous and exogenous (e.g., Posner, 1980 ; Jonides, 1981 ; Weichselgartner and Sperling, 1987 ; Müller and Rabbitt, 1989 ; Nakayama and MacKeben, 1989 ; Cheal and Lyon, 1991 ; Egeth and Yantis, 1997 ). Endogenous attention is a relatively slow process under voluntary control and its allocation to stimuli is flexible. It can be allocated to a static stimulus (fixed retinotopic location) as well as dynamic stimuli when we track for example a moving stimulus (changing retinotopic location; Pylyshyn and Storm, 1988 ). Exogenous attention is a relatively fast reflexive component. It has been shown that exogenous attention can also be deployed non-retinotopically according to the motion and motion-based perceptual of grouping of stimuli (Boi et al., 2011 ; Theeuwes et al., 2013 ; Gonen et al., 2014 ). Hence both endogenous and exogenous attention can operate in terms of retinotopic and non-retinotopic motion-grouping based coordinate systems and can control information flow from SM to STM. In Section Sequential Metacontrast: Non-retinotopic Information Storage And Processing, we discussed findings from sequential metacontrast with cueing, indicating that feature integration within a motion stream does not depend on the spatial allocation or the timing of attention. In the same study, we have also investigated a more complex stimulus where two motion streams merge to form a more complex Gestalt (Figure 9 ). The stimulus consisted of four motion streams, two moving rightward and two moving leftward. Two of these streams merged at a common point. When observers were asked to report the vernier offset of this common point, the outcome did depend on the allocation of attention Figure 9 ). The vernier offset in the attended stream dominated the outcome (Otto et al., 2010a ). To summarize, nrSM has both pre-attentive and attentive components. Storage and integration of information within motion streams are pre-attentive whereas storage and integration of information across motion streams that merge (i.e., grouped into a more complex Gestalt) are flexible and depend on attention. This modulatory effect of attention on non-retinotopic integration of information may be related to the findings of Cavanagh et al. ( 2008 ) who showed that attributes of a moving stimulus which is spatio-temporally embedded in a distractor stimulus can be integrated non-retinotopically when the moving stimulus is tracked by attention. A difference between Cavanagh et al. study and ours is that in their study color and motion attributes integrated non-retinotopically whereas letter and digit shapes did not. In our study, we showed integration for vernier offsets, which would imply integration for shapes. Future studies are needed to clarify this difference.
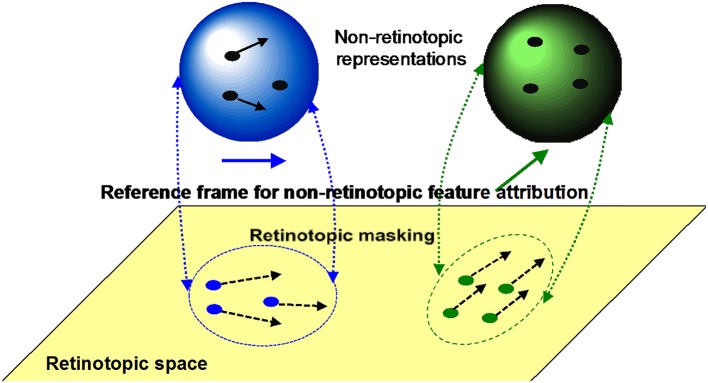
The operation of nr SM . First, a motion analysis is carried out in the retinotopic areas and stimuli are grouped according to Gestalt grouping principles (e.g., common fate). In this example, two groups are formed, the rightward moving blue dots, and upward moving green dots. For each group, a common motion is extracted as the reference-frame and stimuli are transferred into non-retinotopic representations according to this reference-frame. The non-retinotopic representations are depicted at the top of the figure. nr SM stores information within this non-retinotopic representation.
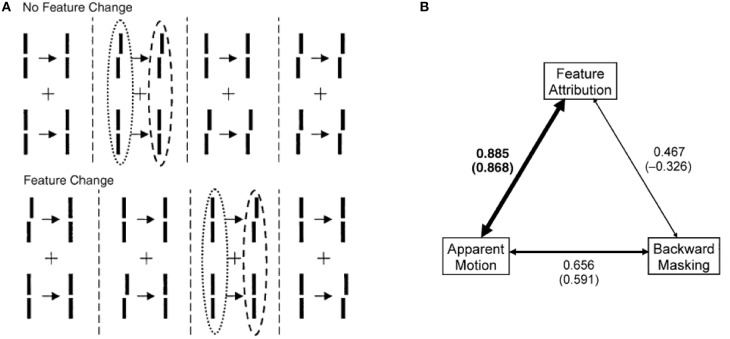
Stimuli consisted of a first frame containing two verniers at the left of the fixation, followed after an ISI by a second frame where the two verniers were presented to the right of the fixation cross . Vernier offsets were introduced so that either there was no change between the two frames, as illustrated by the examples at the top of (A) , or there was a change from the first to the second frame (bottom of A ). In separate session, we asked observers (i) to rate the strength of smooth apparent motion (motion task), (ii) to report whether the upper and lower verniers in the first frame had the same offset or not (masking task), or (iii) to report whether the upper and lower verniers in the second frame had the same offset or not (feature attribution task). The feature attribution task reflects the operation of nr SM. (B) Correlations between motion, masking, and feature attribution. The width of the arrows is directly proportional to strength of the correlations. For each pair of variables, bivariate correlations are given without parentheses and partial correlations are given in parentheses. Only the correlation between feature attribution ( nr SM) and motion was significantly larger than zero (indicated by boldface). From Breitmeyer et al. ( 2008 ).
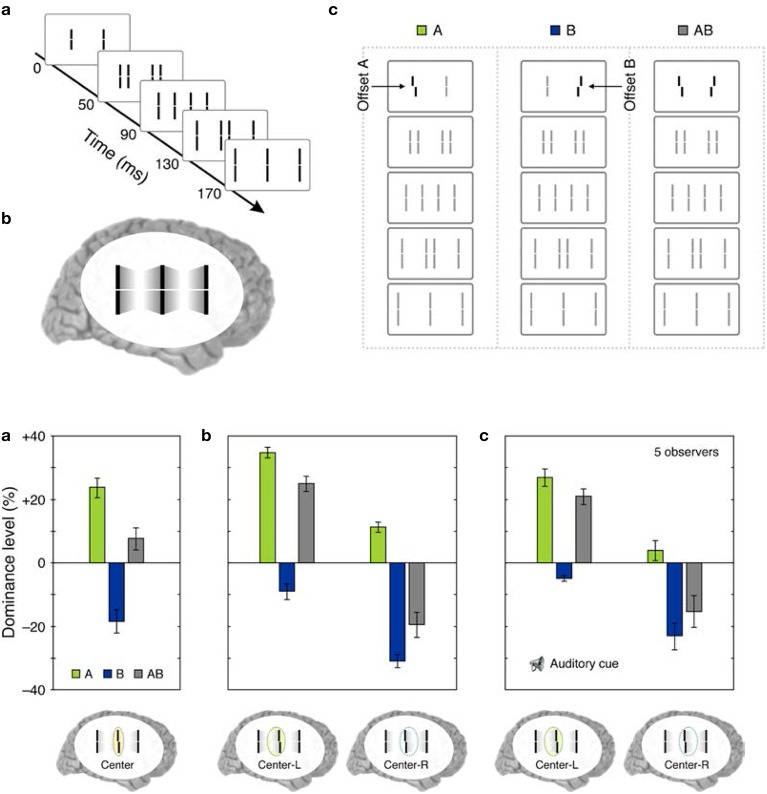
Top panel: (a) The stimulus consisted of four motion streams emerging from two elements . Two of these motion streams merged to a common element in the last frame. (b) The percept. (c) A probe-vernier was inserted to the left element in the first frame (A), an anti-vernier was inserted to the right element in the first frame (B), or both the vernier and the anti-vernier were present (AB). Bottom panel: (a) Observers were instructed to focus their attention on the common central element in the last frame and report the perceived vernier offset for this element. In conditions A and B, the probe-vernier and anti-vernier dominate responses, respectively, as indicated by positive and negative values of dominance level. In condition AB, the dominance level equals approximately to the sum of individual dominance levels of A and B. (b) Observers were instructed to report the perceived vernier offset of the central element in the last frame while focusing their attention either on the left (Center-L) or right (Center-R) motion streams. Focusing attention on the left frame increases the dominance of the probe-vernier and decreases the dominance of the anti-vernier. Focusing attention on the right stream has the opposite effect. (c) The same attentional modulation as in (b) is observed even when the stream to focus attention was indicated by an auditory cue is delivered with a cue-stimulus onset asynchrony of 500 ms, i.e., more than 300 ms after the two motion streams merged to the common element. Adapted from Otto et al., 2010a ; the Association for Research in Vision and Ophthalmology (© ARVO).
Paradoxes of retinotopic sensory memory revisited
Having introduced the new model, we can now compare it to the standard model containing only r SM and discuss what it predicts for the data that have been problematic for r SM.
Anorthoscopic perception
One possible way r SM can account for anorthoscopic perception is to assume that the observers eyes move and hence different parts of the figure fall in different parts of the retina, building up over time a retinotopic image of the stimulus which can be stored by r SM. In fact, this is the “retinal painting” hypothesis which was put forth by von Helmholtz ( 1867 ). While it is possible to store an anorthoscopic stimulus in r SM via eye movements through gradually built-up retinotopic representations, numerous studies showed that anorthoscopic perception does occur in the absence of eye movements, i.e., without retinal painting, for example by moving stimuli in opposite directions (since the eyes cannot pursue simultaneously both stimuli) or by carefully monitoring eye movements during anorthoscopic perception (McCloskey and Watkins, 1978 ; Rock, 1981 ; Morgan et al., 1982 ; Fujita, 1990 ; Sohmiya and Sohmiya, 1992 , 1994 ; Nishida, 2004 ; Fendrich et al., 2005 ; Rieger et al., 2007 ). In the absence of eye movements, since the stimulus moving behind the slit activates the same retinotopic area successively in time, these successive stimulations should be integrated together and stored in r SM as a meaningless blend of different parts. To explain anorthoscopic percepts, Parks ( 1965 ) proposed a non-retinotopic memory using the “time-of-arrival coding.” The storage in this memory is based, not on retinotopic coordinates, but on temporal coordinates with each stimulus part assuming as its coordinate its time-of-arrival. However, time-of-arrival theory was rejected by experimental studies that used a stimulus moving to the right and its mirror-image version moving to the left (McCloskey and Watkins, 1978 ; Sohmiya and Sohmiya, 1992 , 1994 ). Figure 10 shows the stimulus. Two mirror-image symmetric triangular shapes composed of dots travel in opposite directions through the slit. Their timing and speed is arranged so that equivalent parts of the upper and lower triangles pass through the slit simultaneously. If time-of-arrival were the encoding principle in non-retinotopic memory, the upper and lower stimuli should appear identical since the arrival-times of their parts are identical 4 . However, observers report, not two identical triangles, but two mirror-image symmetric triangles.
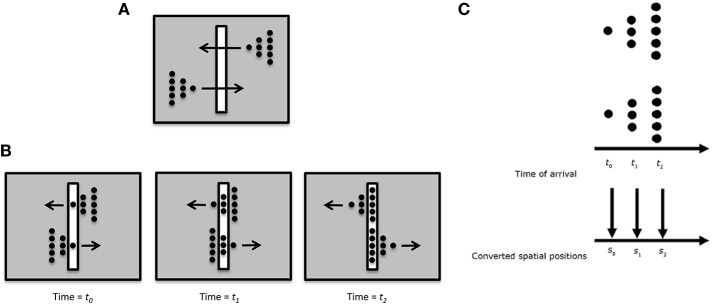
(A) Stimulus configuration used to test the “retinal painting” account based on r SM and a non-retinotopic explanation based on “time-of-arrival” coding. (B) Equivalent parts of the upper and lower stimuli traverse through the slit synchronously. Since an eye movement will generate the same retinal painting for the upper and lower stimuli, the retinal painting account predicts that observers will perceive the same shape for the upper and lower stimuli. (C) The time-of-arrival account converts the arrival times to spatial positions. Since the equivalent parts of the upper and lower stimuli ae synchronized, the time-of-arrival account also predicts that observers will perceive the same shape for the upper and lower stimuli. Contrary to these predictions, observers perceive two mirror-image triangles.
We have proposed an alternative non-retinotopic process to explain anorthoscopic percepts (Öğmen, 2007 ; Aydin et al., 2008 , 2009 ;. The aforementioned experiment suggests that the critical variable is not the time-of-arrival of the stimulus but it is its direction of motion. As illustrated in Figure 7 , we suggested that at a first stage motion vectors are extracted within the retinotopic slit region and these motion vectors (and not the time-of-arrival) serve as the reference-frame for nr SM. Accordingly, for the upper triangle, the leftward motion will be the reference-frame; whereas for the lower triangle rightward motion will be the reference frame. This allows the recovery and storage of the shape information into nr SM. Moreover, we made a novel prediction from our theory that shape distortions observed in anorthoscopic stimuli should be the result of differences in the perceived speeds of different parts of the stimuli. Our data provided support for this prediction (Aydin et al., 2008 ).
Hence, during anorthoscopic perception information is conveyed through nr SM, providing a solution to the paradox of anorthoscopic perception.
How the visual system controls deleterious effects of r SM: motion deblurring
As mentioned before, the visible component (i.e., visible persistence) of the retinotopic sensory memory retains information for about 120 ms under normal viewing conditions (Coltheart, 1980 ). Based on this estimate, one would expect moving objects to appear highly smeared; however, our typical perception is relatively sharp and clear (e.g., Ramachandran et al., 1974 ; Burr, 1980 ; Hogben and Di Lollo, 1985 ; Castet, 1994 ; Bex et al., 1995 ; Westerink and Teunissen, 1995 ; Burr and Morgan, 1997 ; Hammett, 1997 ). This leads to two fundamental questions: (i) how does the visual system generate and store clear percepts if no meaningful information is conveyed by the SM stage of the modal model? and (ii) how does it avoid motion blur; i.e., how does the visual system control deleterious effects of r SM [Q2 in Section Sensory (Iconic) Memory]?
Burr and colleagues measured the perceived extent of motion blur produced by a field of moving dots and showed that it increases as a function of exposure duration up to 40 ms after which it decreases, a phenomenon called motion deblurring (Burr, 1980 ; Burr and Morgan, 1997 ). They proposed that spatiotemporally-oriented receptive-fields of motion mechanisms can account for motion deblurring since these receptive fields can collect information along the motion path of the object (Burr and Morgan, 1997 ). Hence, according to this theory, the computation of form for moving objects is carried out by motion mechanisms. To clarify this concept, consider first the space-time diagram shown in Figure 11A . The red line represents a static stimulus (since its position with respect to the horizontal space-axis is fixed). It will activate a receptive field collecting information from this position over time (depicted by the solid rectangle). Neighboring receptive fields (depicted by dashed rectangles) will not be activated since the stimulus does not fall within their “space-time window.” Hence, the activity generated by the static stimulus will be spatially localized without any blur. A stimulus moving with a constant speed can be represented by an oriented line in the space-time diagram (the red line in Figure 11B ). Motion-sensitive neurons can be described by spatio-temporally oriented receptive fields (Adelson and Bergen, 1985 ). In terms of motion mechanisms that are tuned to the velocity of the stimulus, only one motion mechanism will be activated. Since the case in Figure 11B is equivalent to the static case in Figure 11A with a rotation, Burr and colleagues argued that the stimulus will not generate motion blur provided that it remains with the receptive field of the matching motion detector (the solid rectangle in Figure 11B ) to sufficiently activate it. However, this theory fails to explain the following: As depicted in Figure 11C , mechanisms whose receptive fields are not aligned with the motion path of the object (e.g., motion detectors tuned to different speeds than the speed of the moving object; mechanisms that are not tuned to motion) will be partially activated by the moving object and will generate extensive blur. This theory cannot explain how this blur is avoided by the visual system. Furthermore, since object trajectories can be arbitrarily complex, a fixed set of oriented receptive fields cannot guarantee that a match between object motion and receptive field profile would occur in general (Figure 11D ).
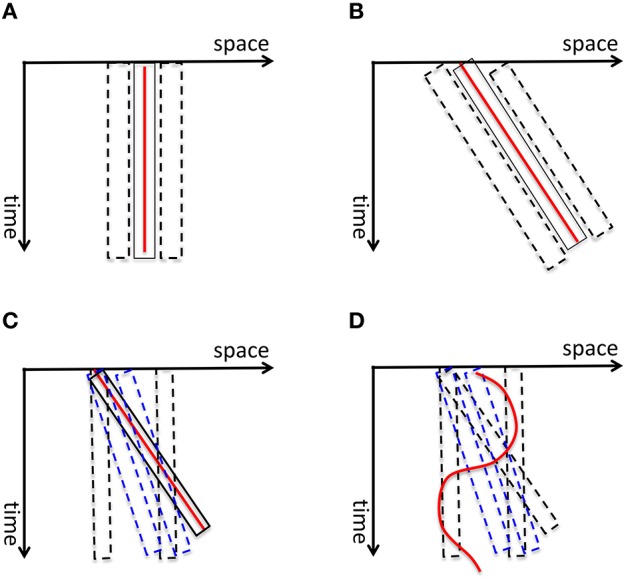
(A) The red vertical line depicts a static stimulus. It activates a receptive field, depicted by the solid rectangle, positioned at the location of the stimulus. The neighboring receptive fields (depicted by dashed rectangles) are not activated. Hence the activity generated by the static stimulus is spatially localized, i.e., without blur. (B) A stimulus moving with a constant speed is depicted by the red line in the space-time diagram. According to Burr and colleagues' theory of motion deblurring, a mechanism which is tuned to the speed of the stimulus, depicted by the solid rectangle, will integrate the stimulus along its motion path and will not generate motion blur. This is because, as in the static case in (A), the neighboring receptive fields are not activated. (C) However, other mechanisms, such as static mechanisms depicted by dashed black rectangles and motion mechanisms whose speed tuning is different than the speed of the stimulus (depicted by blue dashed lines), will be activated by the stimulus only partially and will generate residual activity that persists at their retinotopic location, hence motion blur. Bur and colleagues theory does not account how this motion blur is avoided. (D) Moreover, for a stimulus that moves with a more complex motion trajectory, no single mechanism will be able to integrate its information along its motion path, unless motion detectors for all possible motion paths are pre-wired at all possible retinotopic locations.
Kahneman et al. ( 1992 ) proposed the object-file theory to explain how the attributes of moving objects can be computed. According to this theory, an “object-file” is opened and the attributes of the moving object are inserted into this file. Since this insertion can take place over multiple retinotopic locations over the motion path, the theory could in principle provide an answer to question Q1. However, the theory gives no details, nor mechanisms to explain how object files are opened and information is inserted over the motion pathway. The theory does not answer questions Q2 and Q3 either.
Contrary to the predictions of these two theories, it has been long known that isolated targets in motion do exhibit extensive blur (Bidwell, 1899 ; McDougall, 1904 ; Dixon and Hammond, 1972 ; Farrell, 1984 ; Di Lollo and Hogben, 1985 ; Farrell et al., 1990 ). In order to reconcile the apparently contradictory observations of motion deblurring for a field of moving dots and extensive blur for isolated moving targets, we conducted experiments where we showed that (data, modeling, and review: Chen et al., 1995 ; Purushothaman et al., 1998 ; Öğmen, 2007 ): (1) isolated targets moving on a uniform background are perceived with extensive motion blur; (2) the presence of spatio- temporally proximal stimuli can reduce the spatial extent of perceived motion blur (motion deblurring); (3) motion mechanisms cannot account for motion deblurring; (4) metacontrast mechanisms can account for motion deblurring. Hence, to put these results in the context of our model in Figure 6 , when isolated targets are in motion r SM becomes active and its side-effect, motion blur, is perceived. On the other hand, in the presence of spatiotemporally proximal stimuli, masking “turns off” r SM and no motion blur is perceived. Thus, the answer to the question Q2 is: visual masking. While earlier analyses also acknowledged that visual masking can turn r SM off under most ecologically valid viewing conditions, this observation led to a paradox: If the contents of r SM are suppressed during natural viewing, no information can be conveyed to WM and LTM, making the whole memory system inoperational under normal viewing conditions! Our theory offers a solution to this paradox by suggesting that information is conveyed to STM/WM and LTM through nr SM.
What purpose does r SM serve?
As mentioned in the previous section, data showing that isolated moving-targets do generate motion blur indicate that r SM cannot be completely removed from SM, but its deleterious effects for dynamic viewing conditions are in general controlled by visual masking mechanisms. This leads to a more general question: If r SM cannot be eliminated from SM, is it a simple side-effect or does it serve a purpose? Ecological viewing consists of periods of fixations, saccades, pursuit, and vergence eye movements. The head and the body of the observer can be also in movement and vestibulo-ocular movements can compensate for some but not all retinotopic motions generated by these movements. For example, when the observer moves, the eyes may reflexively compensate for these movements to keep their positions on the fixation point of interest, thereby stabilizing the fixation point. However, observer's movements can also generate motion parallax for the rest of the scene and the amount of motion for different parts of the stimulus depends on the depth of objects relative to the observer. Hence, under normal viewing conditions, the retinotopic stimulus typically contains both static and dynamic components. As we have noted earlier (Footnote 2), static stimuli have a null velocity vector and their reference-frame is equivalent to a retinotopic reference frame. From a mechanistic point of view, if nr SM uses the activities of motion detectors to synthesize its reference-frame, in the case of static stimuli, there will be no motion-detector activity to generate the reference-frame. Hence to store information about static stimuli, a memory component that is directly anchored in retinotopic coordinates is needed and this memory component is r SM. Within nr SM, there can be multiple reference-frames deployed at different parts of the visual field depending on the motion patterns across the visual field. Hence, our theory suggests that sensory memory operates according to retinotopic motion patterns and r SM is a special case with a reference–frame corresponding to the null velocity. From this perspective, information can flow simultaneously from r SM and nr SM, the former carrying out the information about fixated stimuli and the rest of the scene which is static with respect to the fixated stimuli, whereas nr SM conveys information about objects that are in relative motion with respect to the fixation target.
Sensory memory was discovered in 1960s and, by the end of the decade, it became an important and integral part of the modal model of human memory. However, about two decades after its discovery, Haber placed it on a death-bed and suggested that the concept should be removed from textbooks (Haber, 1983 ). The inability of the sensory memory to operate under normal viewing conditions not only challenged any role it may have in information processing, but also positioned it as a “road block” to information flow from external inputs to the rest of the modal model. However, during the last decade, evidence has been accumulating on non-retinotopic processing for various stimulus attributes such as form (Nishida, 2004 ; Öğmen et al., 2006 ; Otto et al., 2006 ; Öğmen and Herzog, 2010 ), luminance (Shimozaki et al., 1999 ), color (Nishida et al., 2007 ), size (Kawabe, 2008 ), and motion (Boi et al., 2009 ; Noory et al., 2015a ). We suggest that this non-retinotopic processing extends to sensory memory in the form of non-retinotopic sensory memory ( nr SM). Furthermore, we have also shown that attention, a key process in the transfer of information from SM to STM, also operates on motion-based non-retinotopic coordinates (Boi et al., 2009 , 2011 ). Based on these findings, we proposed here a new model for SM and discussed how it can resolve the paradoxes that stem from the Achilles' heel of the traditional SM, namely its retinotopic basis.
The traditional SM has been conceptualized as a low-level, image-like representation. However, our results and model suggest that grouping operations already take place in SM. One can also trace the roots of processing stages, such as object permanence and invariance, hitherto thought to take place at higher levels, already in SM. Having a flexible motion-based reference-frame makes this memory position-invariant. Moreover, the ability to carry information across occlusions plays a key role in achieving object permanence. Having these properties already at the SM level does make sense if one considers the ecology of vision. Gestalt psychologists have long argued that atomistic approaches, which build complex percepts by gradually combining simpler ones, cannot handle the complexity of our visual environment and grouping operations need to take place early on. Gibsonian ecological optics (Gibson, 1979 ) emphasizes the importance of motion in a natural environment. Duncker's ( 1929 ) and Johansson's ( 1975 ) work provided several examples of relativity of motion and the underlying motion-based reference frames (reviews: Mack, 1986 ; Öğmen and Herzog, 2015 ). Our new model for sensory memory combines these concepts and suggests how memory systems can be interfaced to our natural environment.
Author contributions
HO, Developed the theory; wrote the original manuscript draft. MH, Developed the theory; read and commented on the original manuscript draft.
Conflict of interest statement
The authors declare that the research was conducted in the absence of any commercial or financial relationships that could be construed as a potential conflict of interest.
Acknowledgments
MH is supported by the Swiss National Science Foundation (SNF) Project “Basics of visual processing: from retinotopic encoding to non-retinotopic representations.”
1 More recently, a new type of STM, called fragile STM has been proposed (Sligte et al., 2008 ; Pinto et al., 2013 ). It has been suggested that fragile STM takes an intermediate position between SM and STM. Whether this is a memory component genuinely distinct from STM is under debate (e.g., Matsukura and Hollingworth, 2011 ; Makovski, 2012 ). In general, fragile STM seems to share the properties of STM, rather than SM. However, because it has not been tested with moving stimuli, it is difficult to compare it with the non-retinotopic sensory memory that we discuss here. We can draw, however, two important distinctions, between non-retinotopic memory that we discuss and fragile STM: Fragile STM is very sensitive to attention and cueing; but as we discuss in Sections Sequential Metacontrast: Non-Retinotopic Information Storage and Processing and A New Conceptualization of Human Sensory Memory, the role of attention in non-retinotopic memory depends on the stimulus configuration. Second, interference (or masking) happens to the contents of fragile STM when similar stimuli are presented at the same locations. As we discuss in Section Sequential Metacontrast: Non-retinotopic Information Storage and Processing, even though stimulus can be completely masked, its informational contents are robust in non-retinotopic memory.
2 A distinction has been made between visible persistence vs. informational persistence (Coltheart, 1980 ). Some authors use the term iconic memory only for the informational persistence component of visual SM, whereas others use for both visual and informational components. We use the terms Sensory Memory and iconic memory to include both visible and informational persistence components.
3 Note that for static stimuli and static observer, the velocity is 0 and hence “motion-based” reference-frame with a null velocity vector becomes identical to a retinotopic reference-frame. However, from a mechanistic point of view, if nr SM uses the activities of motion detectors to synthesize its reference-frame, in the case of static stimuli, there will be no motion-detector activity to generate the reference-frame. Hence to store information about static stimuli, a memory component which is directly anchored in retinotopic coordinates ( r SM) is needed. This is analogous to on and off channels in the visual system. Although these channels can be viewed as part of a single continuum of contrast, computationally, they involve different operations and are represented by separate distinct channels.
4 Note that there is a slight difference in the way the individual disks arrive within the slit; according to time-of-arrival coding, the disks of the top triangle will be constructed from left to right whereas the disks of the bottom triangle will be constructed from right to left. However, in both cases, the same global triangular shape will emerge from these disks regardless whether the individual disks are constructed from left to right or right to left.
- Adelson E. H., Bergen J. R. (1985). Spatiotemporal energy models for the perception of motion. J. Opt. Soc. Am. A 2, 284–299. 10.1364/JOSAA.2.000284 [ DOI ] [ PubMed ] [ Google Scholar ]
- Agaoglu M. N., Herzog M. H., Öğmen H. (2012). Non-retinotopic feature processing in the absence of retinotopic spatial layout and the construction of perceptual space from motion. Vision Res. 71, 10–17. 10.1016/j.visres.2012.08.009 [ DOI ] [ PMC free article ] [ PubMed ] [ Google Scholar ]
- Atkinson R. C., Shiffrin R. M. (1968). Human memory: a proposed system and its control processes, in The Psychology of Learning and Motivation: Advances in Research and Theory, ed Spence K. W. (New York, NY: Academic Press; ) 89–195. [ Google Scholar ]
- Averbach E., Coriell A. (1961). Short-term memory in vision. Bell Syst. Tech. J. 40, 309–328. 10.1002/j.1538-7305.1961.tb03987.x [ DOI ] [ Google Scholar ]
- Averbach E., Sperling G. (1961). Short-term storage of information in vision, in Information Theory, ed Cherry C. (London: Butterworth; ), 196–211. [ Google Scholar ]
- Aydin M., Herzog M. H., Öğmen H. (2008). Perceived speed differences explain apparent compression in slit viewing. Vision Res. 48, 1603–1612. 10.1016/j.visres.2008.04.020 [ DOI ] [ PubMed ] [ Google Scholar ]
- Aydin M., Herzog M. H., Öğmen H. (2009). Shape distortions and Gestalt grouping in anorthoscopic perception. J. Vis. 9, 8.1–8.8. 10.1167/9.3.8 [ DOI ] [ PMC free article ] [ PubMed ] [ Google Scholar ]
- Bachmann T. (1994). Psychophysiology of Visual Masking: The Fine Structure of Conscious Experience. New York, NY: Nova Science Publishers. [ Google Scholar ]
- Baddeley A. (2007). Working Memory, Thought, and Action. Oxford: Oxford University Press; 10.1093/acprof:oso/9780198528012.001.0001 [ DOI ] [ Google Scholar ]
- Bex P. J., Edgar G. K., Smith A. T. (1995). Sharpening of blurred drifting images. Vision Res. 35, 2539–2546. 10.1016/0042-6989(95)00060-D [ DOI ] [ PubMed ] [ Google Scholar ]
- Bidwell S. (1899). Curiosities of Light and Sight. London: Swan Sonnenschein. [ Google Scholar ]
- Boi M., Ogmen H., Herzog M. H. (2011). Motion and tilt after effects occur largely in retinal, not in object coordinates, in the Ternus-Pikler display. J. Vis. 11, 1–11. 10.1167/11.3.7 [ DOI ] [ PMC free article ] [ PubMed ] [ Google Scholar ]
- Boi M., Öğmen H., Krummenacher J., Otto T. U., Herzog M. H. (2009). A (fascinating) litmus test for human retino- vs. non-retinotopic processing. J. Vis. 9:5. 10.1167/9.13.5 [ DOI ] [ PMC free article ] [ PubMed ] [ Google Scholar ]
- Breitmeyer B. G., Öğmen H. (2000). Recent models and findings in backward visual masking: a comparison, review, and update. Percept. Psychophys. 62, 1572–1595. 10.3758/BF03212157 [ DOI ] [ PubMed ] [ Google Scholar ]
- Breitmeyer B. G., Öğmen H. (2006). Visual Masking: Time Slices Through Conscious and Unconscious Vision, 2nd Edn. Oxford, UK: Oxford University Press. [ Google Scholar ]
- Breitmeyer B. G., Herzog M. H., Öğmen H. (2008). Motion, not masking, provides the medium for feature attribution. Psychol. Sci. 19, 823–829. 10.1016/j.ptsp.2015.10.005 [ DOI ] [ PubMed ] [ Google Scholar ]
- Breitmeyer B. G., Kropfl W., Julesz B. (1982). Tex existence and role of retinotopic and spatiotopic forms of visual persistence. Acta Psychol. 52, 175–196. 10.1016/0001-6918(82)90007-5 [ DOI ] [ PubMed ] [ Google Scholar ]
- Burr D. (1980). Motion smear. Nature 284, 164–165. 10.1038/284164a0 [ DOI ] [ PubMed ] [ Google Scholar ]
- Burr D. C., Morgan M. J. (1997). Motion deblurring in human vision. Proc. Soc. Lond. B 264, 431–436. 10.1098/rspb.1997.0061 [ DOI ] [ PMC free article ] [ PubMed ] [ Google Scholar ]
- Castet E. (1994). Effect of the ISI on the visible persistence of a stimulus in apparent motion. Vision Res. 34, 2103–2114. 10.1016/0042-6989(94)90320-4 [ DOI ] [ PubMed ] [ Google Scholar ]
- Cavanagh P., Holcombe A. O., Chou W. (2008). Mobile computation: spatiotemporal integration of the properties of objects in motion. J. Vis. 8, 1–23. 10.1167/8.12.1 [ DOI ] [ PMC free article ] [ PubMed ] [ Google Scholar ]
- Cheal M., Lyon D. R. (1991). Central and peripheral precuing of forced-choice discrimination. Q. J. Exp. Psychol. 43A, 859–880. 10.1080/14640749108400960 [ DOI ] [ PubMed ] [ Google Scholar ]
- Chen S., Bedell H. E., Öğmen H. (1995). A target in real motion appears blurred in the absence of other proximal moving targets. Vision Res. 35, 2315–2328. 10.1016/0042-6989(94)00308-9 [ DOI ] [ PubMed ] [ Google Scholar ]
- Coltheart M. (1980). Iconic memory and visible persistence. Percept. Psychophys. 27, 183–228. 10.3758/BF03204258 [ DOI ] [ PubMed ] [ Google Scholar ]
- Davidson M. L., Fox M. J., Dick A. O. (1973). Effect of eye movements on backward masking and perceived location. Percept. Psychophys. 14, 110–116. 10.3758/BF03198624 [ DOI ] [ Google Scholar ]
- Di Lollo V., Hogben J. H. (1985). Suppression of visible persistence. J. Exp. Psychol. 11, 304–316. 10.1037/0096-1523.11.3.304 [ DOI ] [ PubMed ] [ Google Scholar ]
- Dixon N. F., Hammond E. J. (1972). The attenuation of visual persistence. Br. J. Psychol. 63, 243–254. 10.1111/j.2044-8295.1972.tb02107.x [ DOI ] [ PubMed ] [ Google Scholar ]
- Duncker K. (1929). Uber induzierte Bewegung. Psychol. Forsch. 22, 180–259. 10.1007/BF02409210 [ DOI ] [ Google Scholar ]
- Egeth H. E., Yantis S. (1997). Visual attention: control, representation, and time course. Annu. Rev. Psychol. 48, 269–297. 10.1146/annurev.psych.48.1.269 [ DOI ] [ PubMed ] [ Google Scholar ]
- Farrell J. E. (1984). Visible persistence of moving objects. J. Exp. Psychol. 10, 502–511. 10.1037/0096-1523.10.4.502 [ DOI ] [ PubMed ] [ Google Scholar ]
- Farrell J. E., Pavel M., Sperling G. (1990). Visible persistence of stimuli in stroboscopic motion. Vision Res. 30, 921–936. 10.1016/0042-6989(90)90058-S [ DOI ] [ PubMed ] [ Google Scholar ]
- Fendrich R., Rieger J. W., Heinze H.-J. (2005). The effect of retinal stabilization on anorthoscopic percepts under free-viewing conditions. Vision Res. 45, 567–582. 10.1016/j.visres.2004.09.025 [ DOI ] [ PubMed ] [ Google Scholar ]
- Fujita N. (1990). Three-dimensional anorthoscopic perception. Perception 19, 767–771. 10.1068/p190767 [ DOI ] [ PubMed ] [ Google Scholar ]
- Gibson J. J. (1979). The Ecological Approach to Visual Perception. Boston, MA: Houghton Mifflin. [ Google Scholar ]
- Gonen F. F., Hallal H., Öğmen H. (2014). Facilitation by exogenous attention for static and dynamic gestalt groups. Atten. Percept. Psychophys. 76, 1709–1720. 10.3758/s13414-014-0679-2 [ DOI ] [ PubMed ] [ Google Scholar ]
- Griffin I. C., Nobre A. C. (2003). Orienting attention to locations in internal representations. J. Cogn. Neurosci. 15, 1176–1194. 10.1162/089892903322598139 [ DOI ] [ PubMed ] [ Google Scholar ]
- Haber R. N. (1983). The impending demise of the icon: a critique of the concept of iconic storage in visual information processing. Behav. Brain Sci. 6, 1–54. [ Google Scholar ]
- Hammett S. T. (1997). Motion blur and motion sharpening in the human visual system. Vision Res. 37, 2505–2510. 10.1016/S0042-6989(97)00059-X [ DOI ] [ PubMed ] [ Google Scholar ]
- Hogben J. H., Di Lollo V. (1985). Suppression of visible persistence in apparent motion. Percept. Psychophys. 38, 450–460. 10.3758/BF03207176 [ DOI ] [ PubMed ] [ Google Scholar ]
- Hollingworth A., Maxcey-Richard A. M. (2013). Selective maintenance in visual working memory does not require sustained visual attention. J. Exp. Psychol. 39, 1047–1058. 10.1037/a0030238 [ DOI ] [ PMC free article ] [ PubMed ] [ Google Scholar ]
- Irwin D. E., Brown J. S., Sun J.-S. (1988). Visual masking and visual integration across saccadic eye movements. J. Exp. Psychol. 117, 276–287. 10.1037/0096-3445.117.3.276 [ DOI ] [ PubMed ] [ Google Scholar ]
- Irwin D. E., Yantis S., Jonides J. (1983). Evidence against visual integration across saccadic eye movements. Percept. Psychophys. 34, 49–57. [ DOI ] [ PubMed ] [ Google Scholar ]
- Johansson G. (1975). Visual motion perception. Sci. Am. 232, 76–88. 10.1007/s00221-014-3959-0 [ DOI ] [ PubMed ] [ Google Scholar ]
- Jonides J. (1981). Voluntary vs. Automatic control over the mind's eye's movement, in Attention and Performance IX, eds Long J., Baddeley A. (Hillsdale, MI: Erlbaum; ), 187–203. [ Google Scholar ]
- Jonides J., Irwin D. E., Yantis S. (1982). Integrating visual information from successive fixations. Science 215, 192–194. 10.1126/science.7053571 [ DOI ] [ PubMed ] [ Google Scholar ]
- Jonides J., Irwin D. E., Yantis S. (1983). Failure to integrate information from successive fixations. Science 222, 188. 10.1126/science.6623072 [ DOI ] [ PubMed ] [ Google Scholar ]
- Kahneman D., Treisman A., Gibbs B. J. (1992). The reviewing of object files: object-specific integration of information. Cogn. Psychol. 24, 175–219. 10.1016/0010-0285(92)90007-o [ DOI ] [ PubMed ] [ Google Scholar ]
- Kawabe T. (2008). Spatiotemporal feature attribution for the perception of visual size. J. Vis. 8, 7.1–7.9. 10.1167/8.8.7 [ DOI ] [ PubMed ] [ Google Scholar ]
- Mack A. (1986). Perceptual aspects of motion in the frontal plane, in Handbook of Perception and Human Performance, eds Boff K. R., Kaufman L., Thomas J. P. (New York, NY: John Wiley and Sons; ), 17.1–17.38. [ Google Scholar ]
- Makovski T. (2012). Are multiple visual short-term memory storages necessary to explain the retro-cue effect? Psychon. Bull. Rev. 19, 470–476. 10.3758/s13423-012-0235-9 [ DOI ] [ PubMed ] [ Google Scholar ]
- Matsukura M., Hollingworth A. (2011). Does visual short-term memory have a high-capacity stage? Psychon. Bull. Rev. 18, 1098–1104. 10.3758/s13423-011-0153-2 [ DOI ] [ PMC free article ] [ PubMed ] [ Google Scholar ]
- McCloskey M., Watkins M. J. (1978). The seeing- more-than-is-there phenomenon: implications for the locus of iconic storage. J. Exp. Psychol. 4, 553–564. 10.1037/0096-1523.4.4.553 [ DOI ] [ PubMed ] [ Google Scholar ]
- McDougall W. (1904). The sensations excited by a single momentary stimulation of the eye. Br. J. Psychol. 1, 78–113. 10.1111/j.2044-8295.1904.tb00150.x [ DOI ] [ Google Scholar ]
- McRae K., Butler B. E., Popiel S. J. (1987). Spatiotopic and retinotopic components of iconic memory. Psychol. Res. 49, 221–227. 10.1007/BF00309030 [ DOI ] [ PubMed ] [ Google Scholar ]
- Morgan M. J., Findlay J. M., Watt R. J. (1982). Aperture viewing: a review and a synthesis. Q. J. Exp. Psychol. 34A, 211–233. 10.1080/14640748208400837 [ DOI ] [ PubMed ] [ Google Scholar ]
- Müller H. J., Rabbitt P. M. (1989). Reflexive and voluntary orienting of visual attention: time course of activation and resistance to interruption. J. Exp. Psychol. 15, 315–330. 10.1037/0096-1523.15.2.315 [ DOI ] [ PubMed ] [ Google Scholar ]
- Nakayama K., MacKeben M. (1989). Sustained and transient compo- nents of focal visual attention. Vision Res. 11, 1631–1647. 10.1016/0042-6989(89)90144-2 [ DOI ] [ PubMed ] [ Google Scholar ]
- Nishida S. (2004). Motion-based analysis of spatial patterns by the human visual system. Curr. Biol. 14, 830–839. 10.1016/j.cub.2004.04.044 [ DOI ] [ PubMed ] [ Google Scholar ]
- Nishida S., Watanabe J., Kuriki I., Tokimoto T. (2007). Human brain integrates colour signals along motion trajectory. Curr. Biol. 17, 366–372. 10.1016/j.cub.2006.12.041 [ DOI ] [ PubMed ] [ Google Scholar ]
- Noory B., Herzog M. H., Öğmen H. (2015a). Spatial properties of non-retinotopic reference frames in human vision. Vision Res. 113, 44–54. 10.1016/j.visres.2015.05.010 [ DOI ] [ PubMed ] [ Google Scholar ]
- Noory B., Herzog M. H., Öğmen H. (2015b). Retinotopy of visual masking and non-retinotopic perception during masking. Atten. Percept. Psychophys. 77, 1263–1284. 10.3758/s13414-015-0844-2 [ DOI ] [ PubMed ] [ Google Scholar ]
- Öğmen H. (2007). A theory of moving form perception: synergy between masking, perceptual grouping, and motion computation in retinotopic and non-retinotopic representations. Adv. Cogn. Psychol. 3, 67–84. 10.2478/v10053-008-0015-2 [ DOI ] [ PMC free article ] [ PubMed ] [ Google Scholar ]
- Öğmen H., Herzog M. H. (2010). The geometry of visual perception: retinotopic and non-retinotopic representations in the human visual system. Proc. IEEE Inst. Electr. Electron. Eng. 98, 479–492. 10.1109/JPROC.2009.2039028 [ DOI ] [ PMC free article ] [ PubMed ] [ Google Scholar ]
- Öğmen H., Herzog M. H. (2015). Apparent motion and reference frames, in Oxford Handbook of Perceptual Organization, ed Wagemans J. (Oxford, UK: Oxford University Press; ), 487–503. [ Google Scholar ]
- Öğmen H., Otto T., Herzog M. H. (2006). Perceptual grouping induces non-retinotopic feature attribution in human vision. Vision Res. 46, 3234–3242. 10.1016/j.visres.2006.04.007 [ DOI ] [ PubMed ] [ Google Scholar ]
- Otto T. U., Öğmen H., Herzog M. H. (2006). The flight path of the phoenix-the visible trace of invisible elements in human vision. J. Vis. 6, 1079–1086. 10.1167/6.10.7 [ DOI ] [ PubMed ] [ Google Scholar ]
- Otto T. U., Öğmen H., Herzog M. H. (2008). Assessing the microstructure of motion correspondences with non-retinotopic feature attribution. J. Vis. 8, 16.1–16.15. 10.1167/8.7.16 [ DOI ] [ PubMed ] [ Google Scholar ]
- Otto T. U., Öğmen H., Herzog M. H. (2009). Feature integration across space, time, and orientation. J. Exp. Psychol. 35, 1670–1686. 10.1037/a0015798 [ DOI ] [ PMC free article ] [ PubMed ] [ Google Scholar ]
- Otto T. U., Öğmen H., Herzog M. H. (2010a). Attention and non-retinotopic feature integration. J. Vis. 10:8. 10.1167/10.12.8 [ DOI ] [ PMC free article ] [ PubMed ] [ Google Scholar ]
- Otto T. U., Öğmen H., Herzog M. H. (2010b). Perceptual learning in a nonretinotopic frame of reference. Psychol. Sci. 21, 1058–1063. 10.1177/0956797610376074 [ DOI ] [ PMC free article ] [ PubMed ] [ Google Scholar ]
- Pantle A., Picciano L. (1976). A multistable movement display: evidence for two separate motion systems in human vision. Science 193, 500–502. 10.1126/science.941023 [ DOI ] [ PubMed ] [ Google Scholar ]
- Parks T. E. (1965). Post-retinal visual storage. Am. J. Psychol. 78, 145–147. 10.2307/1421101 [ DOI ] [ PubMed ] [ Google Scholar ]
- Piéron H. (1935). Le processus du métacontraste. J. Psychol. Normale Pathal. 32, 1–24. [ Google Scholar ]
- Pikler J. (1917). Sinnesphysiologische Untersuchungen. Leipzig: Barth. [ Google Scholar ]
- Pinto Y., Sligte I. G., Shapiro K. L., Lamme V. A. F. (2013). Fragile visual short-term memory is an object-based and location-specific store. Psychon. Bull. Rev. 20, 732–739. 10.3758/s13423-013-0393-4 [ DOI ] [ PubMed ] [ Google Scholar ]
- Plateau J. (1836). Notice sur l'anarthoscope. Bull. Acad. R. Sci. Belles Lett. Bruxelles 3, 7–10. [ Google Scholar ]
- Posner M. I. (1980). Orienting of attention. Q. J. Exp. Psychol. 32, 3–25. 10.1080/13803390903146949 [ DOI ] [ PubMed ] [ Google Scholar ]
- Purushothaman G., Öğmen H., Chen S., Bedell H. E. (1998). Motion deblurring in a neural network model of retino-cortical dynamics. Vision Res. 38, 1827–1842. 10.1016/S0042-6989(97)00350-7 [ DOI ] [ PubMed ] [ Google Scholar ]
- Pylyshyn Z. W., Storm R. W. (1988). Tracking multiple independent targets: evidence for a parallel tracking mechanism. Spat. Vis. 3, 179–197. 10.1163/156856888X00122 [ DOI ] [ PubMed ] [ Google Scholar ]
- Ramachandran V. S., Rao V. M., Vidyasagar T. R. (1974). Sharpness constancy during movement perception. Perception 3, 97–98. 10.1068/p030097 [ DOI ] [ PubMed ] [ Google Scholar ]
- Rayner K., Pollatsek A. (1983). Is visual information integrated across saccades? Percept. Psychophys. 34, 39–48. 10.3758/BF03205894 [ DOI ] [ PubMed ] [ Google Scholar ]
- Rerko L., Souza A. A., Oberauer K. (2014). Retro-cue benefits in working memory without sustained focal attention. Mem. Cogn. 42, 712–728. 10.3758/s13421-013-0392-8 [ DOI ] [ PubMed ] [ Google Scholar ]
- Rieger J. W., Grüschow M., Heinze H.-J., Fendrich R. (2007). The appearance of figures seen through a narrow aperture under free viewing conditions: effects of spontaneous eye motions. J. Vis. 7:10. 10.1167/7.6.10 [ DOI ] [ PubMed ] [ Google Scholar ]
- Ritter M. (1976). Evidence for visual persistence during saccadic eye movements. Psychol. Res. 39, 67–85. 10.1007/BF00308946 [ DOI ] [ PubMed ] [ Google Scholar ]
- Rock I. (1981). Anorthoscopic perception. Sci. Am. 244, 145–153. 10.1038/scientificamerican0381-145 [ DOI ] [ PubMed ] [ Google Scholar ]
- Scharnowski F., Hermens F., Kammer T., Öğmen H., Herzog M. H. (2007). Feature fusion reveals slow and fast memories. J. Cogn. Neurosci. 19, 632–641. 10.1162/jocn.2007.19.4.632 [ DOI ] [ PubMed ] [ Google Scholar ]
- Shimozaki S., Eckstein M. P., Thomas J. P. (1999). The maintenance of apparent luminance of an object. J. Exp. Psychol. Hum. Percept. Perform. 25, 1433–1453. 10.1037/0096-1523.25.5.1433 [ DOI ] [ PubMed ] [ Google Scholar ]
- Sligte I. G., Scholte H. S., Lamme V. A. F. (2008). Are there multiple visual short-term memory stores? PLoS ONE 3:e0001699. 10.1371/journal.pone.0001699 [ DOI ] [ PMC free article ] [ PubMed ] [ Google Scholar ]
- Sohmiya T., Sohmiya K. (1992). Where does an anorthoscopic image appear? Percept. Mot. Skills 75, 707–714. 10.2466/pms.1992.75.3.707 [ DOI ] [ PubMed ] [ Google Scholar ]
- Sohmiya T., Sohmiya K. (1994). What is a crucial determinant in anorthoscopic perception? Percept. Mot. Skills 78, 987–998. 10.2466/pms.1994.78.3.987 [ DOI ] [ PubMed ] [ Google Scholar ]
- Sperling G. (1960). The information available in brief visual presentations. Psychol. Monogr. 74, 1–29. [ Google Scholar ]
- Sun J.-S., Irwin D. E. (1987). Retinal masking during pursuit eye movements: implications for spatiotopic visual persistence. J. Exp. Psychol. 13, 140–145. 10.1037/0096-1523.13.1.140 [ DOI ] [ PubMed ] [ Google Scholar ]
- Ternus J. (1926). Experimentelle Untersuchung über phänomenale Identität. Psychol. Forsch. 7, 81–136. 10.1007/BF02424350 [ DOI ] [ Google Scholar ]
- Theeuwes J., Mathôt S., Grainger J. (2013). Exogenous object- centered attention. Atten. Percept. Psychophys. 75, 812–818. 10.3758/s13414-013-0459-4 [ DOI ] [ PubMed ] [ Google Scholar ]
- Vandenbroucke A. R. E., Sligte I. G., Barrett A. B., Seth A. K., Fahrenfort J. J., Lamme V. A. F. (2014). Accurate metacognition for visual sensory memory representations. Psychol. Sci. 25, 861–873. 10.1177/0956797613516146 [ DOI ] [ PubMed ] [ Google Scholar ]
- van der Heijden A. H. C., Bridgeman B., Mewhort D. J. K. (1986). Is stimulus persistence affected by eye movements? A critique of Davidson, Fox, and Dick (1973). Psychol. Res. 48, 179–181. 10.1007/BF00309166 [ DOI ] [ PubMed ] [ Google Scholar ]
- van Moorselaar D., Olivers C. N. L., Theeuwes J., Lamme V. A. F., Sligte I. G. (2015). Forgotten but not gone: Retro-cue costs and benefits in a double-cueing paradigm suggest multiple states in visual short-term memory. J. Exp. Psychol. Learn. Mem. Cogn. 41, 1755–1763. 10.1037/xlm0000124 [ DOI ] [ PubMed ] [ Google Scholar ]
- von Helmholtz H. (1867). Handbook of Physiological Optics. New York, NY: Dover. Reprint in 1962. [ Google Scholar ]
- Weichselgartner E., Sperling G. (1987). Dynamics of automatic and controlled visual attention. Science 238, 778–780. 10.1126/science.3672124 [ DOI ] [ PubMed ] [ Google Scholar ]
- Westerink J. H. D. M., Teunissen K. (1995). Perceived sharpness in complex moving images. Displays 16, 89–97. 10.1016/0141-9382(95)91178-5 [ DOI ] [ Google Scholar ]
- White C. W. (1976). Visual masking during pursuit eye movements. J. Exp. Psychol. 2, 469–478. 10.1037/0096-1523.2.4.469 [ DOI ] [ PubMed ] [ Google Scholar ]
- Wolfe W., Hauske G., Lupp U. (1978a). How pre-saccadic gratings modify post-saccadic modulation transfer functions. Vision Res. 18, 1173–1179. 10.1016/0042-6989(78)90101-3 [ DOI ] [ PubMed ] [ Google Scholar ]
- Wolfe W., Hauske G., Lupp U. (1978b). Interaction of pre- and post-saccadic patterns having the same co-ordinates in visual space. Vision Res. 20, 117–125. 10.1016/0042-6989(80)90153-4 [ DOI ] [ PubMed ] [ Google Scholar ]
- Wutz A., Melcher D. (2014). The temporal window of individuation limits visual capacity. Front. Psychol. 5:952. 10.3389/fpsyg.2014.00952 [ DOI ] [ PMC free article ] [ PubMed ] [ Google Scholar ]
- Zöllner F. (1862). Über eine neue art anorthoskopischer zerrbilder. Ann. Phys. Chem. 117, 477–484. 10.1002/andp.18621931108 [ DOI ] [ Google Scholar ]
- View on publisher site
- PDF (2.4 MB)
- Collections
Similar articles
Cited by other articles, links to ncbi databases.
- Download .nbib .nbib
- Format: AMA APA MLA NLM
Add to Collections
- Search Menu
Sign in through your institution
- Browse content in Arts and Humanities
- Browse content in Archaeology
- Anglo-Saxon and Medieval Archaeology
- Archaeological Methodology and Techniques
- Archaeology by Region
- Archaeology of Religion
- Archaeology of Trade and Exchange
- Biblical Archaeology
- Contemporary and Public Archaeology
- Environmental Archaeology
- Historical Archaeology
- History and Theory of Archaeology
- Industrial Archaeology
- Landscape Archaeology
- Mortuary Archaeology
- Prehistoric Archaeology
- Underwater Archaeology
- Urban Archaeology
- Zooarchaeology
- Browse content in Architecture
- Architectural Structure and Design
- History of Architecture
- Residential and Domestic Buildings
- Theory of Architecture
- Browse content in Art
- Art Subjects and Themes
- History of Art
- Industrial and Commercial Art
- Theory of Art
- Biographical Studies
- Byzantine Studies
- Browse content in Classical Studies
- Classical History
- Classical Philosophy
- Classical Mythology
- Classical Numismatics
- Classical Literature
- Classical Reception
- Classical Art and Architecture
- Classical Oratory and Rhetoric
- Greek and Roman Papyrology
- Greek and Roman Epigraphy
- Greek and Roman Law
- Greek and Roman Archaeology
- Late Antiquity
- Religion in the Ancient World
- Social History
- Digital Humanities
- Browse content in History
- Colonialism and Imperialism
- Diplomatic History
- Environmental History
- Genealogy, Heraldry, Names, and Honours
- Genocide and Ethnic Cleansing
- Historical Geography
- History by Period
- History of Emotions
- History of Agriculture
- History of Education
- History of Gender and Sexuality
- Industrial History
- Intellectual History
- International History
- Labour History
- Legal and Constitutional History
- Local and Family History
- Maritime History
- Military History
- National Liberation and Post-Colonialism
- Oral History
- Political History
- Public History
- Regional and National History
- Revolutions and Rebellions
- Slavery and Abolition of Slavery
- Social and Cultural History
- Theory, Methods, and Historiography
- Urban History
- World History
- Browse content in Language Teaching and Learning
- Language Learning (Specific Skills)
- Language Teaching Theory and Methods
- Browse content in Linguistics
- Applied Linguistics
- Cognitive Linguistics
- Computational Linguistics
- Forensic Linguistics
- Grammar, Syntax and Morphology
- Historical and Diachronic Linguistics
- History of English
- Language Evolution
- Language Reference
- Language Acquisition
- Language Variation
- Language Families
- Lexicography
- Linguistic Anthropology
- Linguistic Theories
- Linguistic Typology
- Phonetics and Phonology
- Psycholinguistics
- Sociolinguistics
- Translation and Interpretation
- Writing Systems
- Browse content in Literature
- Bibliography
- Children's Literature Studies
- Literary Studies (Romanticism)
- Literary Studies (American)
- Literary Studies (Asian)
- Literary Studies (European)
- Literary Studies (Eco-criticism)
- Literary Studies (Modernism)
- Literary Studies - World
- Literary Studies (1500 to 1800)
- Literary Studies (19th Century)
- Literary Studies (20th Century onwards)
- Literary Studies (African American Literature)
- Literary Studies (British and Irish)
- Literary Studies (Early and Medieval)
- Literary Studies (Fiction, Novelists, and Prose Writers)
- Literary Studies (Gender Studies)
- Literary Studies (Graphic Novels)
- Literary Studies (History of the Book)
- Literary Studies (Plays and Playwrights)
- Literary Studies (Poetry and Poets)
- Literary Studies (Postcolonial Literature)
- Literary Studies (Queer Studies)
- Literary Studies (Science Fiction)
- Literary Studies (Travel Literature)
- Literary Studies (War Literature)
- Literary Studies (Women's Writing)
- Literary Theory and Cultural Studies
- Mythology and Folklore
- Shakespeare Studies and Criticism
- Browse content in Media Studies
- Browse content in Music
- Applied Music
- Dance and Music
- Ethics in Music
- Ethnomusicology
- Gender and Sexuality in Music
- Medicine and Music
- Music Cultures
- Music and Media
- Music and Religion
- Music and Culture
- Music Education and Pedagogy
- Music Theory and Analysis
- Musical Scores, Lyrics, and Libretti
- Musical Structures, Styles, and Techniques
- Musicology and Music History
- Performance Practice and Studies
- Race and Ethnicity in Music
- Sound Studies
- Browse content in Performing Arts
- Browse content in Philosophy
- Aesthetics and Philosophy of Art
- Epistemology
- Feminist Philosophy
- History of Western Philosophy
- Meta-Philosophy
- Metaphysics
- Moral Philosophy
- Non-Western Philosophy
- Philosophy of Language
- Philosophy of Mind
- Philosophy of Perception
- Philosophy of Science
- Philosophy of Action
- Philosophy of Law
- Philosophy of Religion
- Philosophy of Mathematics and Logic
- Practical Ethics
- Social and Political Philosophy
- Browse content in Religion
- Biblical Studies
- Christianity
- East Asian Religions
- History of Religion
- Judaism and Jewish Studies
- Qumran Studies
- Religion and Education
- Religion and Health
- Religion and Politics
- Religion and Science
- Religion and Law
- Religion and Gender
- Religion and Art, Literature, and Music
- Religious Studies
- Browse content in Society and Culture
- Cookery, Food, and Drink
- Cultural Studies
- Customs and Traditions
- Ethical Issues and Debates
- Hobbies, Games, Arts and Crafts
- Lifestyle, Home, and Garden
- Natural world, Country Life, and Pets
- Popular Beliefs and Controversial Knowledge
- Sports and Outdoor Recreation
- Technology and Society
- Travel and Holiday
- Visual Culture
- Browse content in Law
- Arbitration
- Browse content in Company and Commercial Law
- Commercial Law
- Company Law
- Browse content in Comparative Law
- Systems of Law
- Competition Law
- Browse content in Constitutional and Administrative Law
- Government Powers
- Judicial Review
- Local Government Law
- Military and Defence Law
- Parliamentary and Legislative Practice
- Construction Law
- Contract Law
- Browse content in Criminal Law
- Criminal Procedure
- Criminal Evidence Law
- Sentencing and Punishment
- Employment and Labour Law
- Environment and Energy Law
- Browse content in Financial Law
- Banking Law
- Insolvency Law
- History of Law
- Human Rights and Immigration
- Intellectual Property Law
- Browse content in International Law
- Private International Law and Conflict of Laws
- Public International Law
- IT and Communications Law
- Jurisprudence and Philosophy of Law
- Law and Politics
- Law and Society
- Browse content in Legal System and Practice
- Courts and Procedure
- Legal Skills and Practice
- Legal System - Costs and Funding
- Primary Sources of Law
- Regulation of Legal Profession
- Medical and Healthcare Law
- Browse content in Policing
- Criminal Investigation and Detection
- Police and Security Services
- Police Procedure and Law
- Police Regional Planning
- Browse content in Property Law
- Personal Property Law
- Restitution
- Study and Revision
- Terrorism and National Security Law
- Browse content in Trusts Law
- Wills and Probate or Succession
- Browse content in Medicine and Health
- Browse content in Allied Health Professions
- Arts Therapies
- Clinical Science
- Dietetics and Nutrition
- Occupational Therapy
- Operating Department Practice
- Physiotherapy
- Radiography
- Speech and Language Therapy
- Browse content in Anaesthetics
- General Anaesthesia
- Clinical Neuroscience
- Browse content in Clinical Medicine
- Acute Medicine
- Cardiovascular Medicine
- Clinical Genetics
- Clinical Pharmacology and Therapeutics
- Dermatology
- Endocrinology and Diabetes
- Gastroenterology
- Genito-urinary Medicine
- Geriatric Medicine
- Infectious Diseases
- Medical Toxicology
- Medical Oncology
- Pain Medicine
- Palliative Medicine
- Rehabilitation Medicine
- Respiratory Medicine and Pulmonology
- Rheumatology
- Sleep Medicine
- Sports and Exercise Medicine
- Community Medical Services
- Critical Care
- Emergency Medicine
- Forensic Medicine
- Haematology
- History of Medicine
- Browse content in Medical Skills
- Clinical Skills
- Communication Skills
- Nursing Skills
- Surgical Skills
- Browse content in Medical Dentistry
- Oral and Maxillofacial Surgery
- Paediatric Dentistry
- Restorative Dentistry and Orthodontics
- Surgical Dentistry
- Medical Ethics
- Medical Statistics and Methodology
- Browse content in Neurology
- Clinical Neurophysiology
- Neuropathology
- Nursing Studies
- Browse content in Obstetrics and Gynaecology
- Gynaecology
- Occupational Medicine
- Ophthalmology
- Otolaryngology (ENT)
- Browse content in Paediatrics
- Neonatology
- Browse content in Pathology
- Chemical Pathology
- Clinical Cytogenetics and Molecular Genetics
- Histopathology
- Medical Microbiology and Virology
- Patient Education and Information
- Browse content in Pharmacology
- Psychopharmacology
- Browse content in Popular Health
- Caring for Others
- Complementary and Alternative Medicine
- Self-help and Personal Development
- Browse content in Preclinical Medicine
- Cell Biology
- Molecular Biology and Genetics
- Reproduction, Growth and Development
- Primary Care
- Professional Development in Medicine
- Browse content in Psychiatry
- Addiction Medicine
- Child and Adolescent Psychiatry
- Forensic Psychiatry
- Learning Disabilities
- Old Age Psychiatry
- Psychotherapy
- Browse content in Public Health and Epidemiology
- Epidemiology
- Public Health
- Browse content in Radiology
- Clinical Radiology
- Interventional Radiology
- Nuclear Medicine
- Radiation Oncology
- Reproductive Medicine
- Browse content in Surgery
- Cardiothoracic Surgery
- Gastro-intestinal and Colorectal Surgery
- General Surgery
- Neurosurgery
- Paediatric Surgery
- Peri-operative Care
- Plastic and Reconstructive Surgery
- Surgical Oncology
- Transplant Surgery
- Trauma and Orthopaedic Surgery
- Vascular Surgery
- Browse content in Science and Mathematics
- Browse content in Biological Sciences
- Aquatic Biology
- Biochemistry
- Bioinformatics and Computational Biology
- Developmental Biology
- Ecology and Conservation
- Evolutionary Biology
- Genetics and Genomics
- Microbiology
- Molecular and Cell Biology
- Natural History
- Plant Sciences and Forestry
- Research Methods in Life Sciences
- Structural Biology
- Systems Biology
- Zoology and Animal Sciences
- Browse content in Chemistry
- Analytical Chemistry
- Computational Chemistry
- Crystallography
- Environmental Chemistry
- Industrial Chemistry
- Inorganic Chemistry
- Materials Chemistry
- Medicinal Chemistry
- Mineralogy and Gems
- Organic Chemistry
- Physical Chemistry
- Polymer Chemistry
- Study and Communication Skills in Chemistry
- Theoretical Chemistry
- Browse content in Computer Science
- Artificial Intelligence
- Computer Architecture and Logic Design
- Game Studies
- Human-Computer Interaction
- Mathematical Theory of Computation
- Programming Languages
- Software Engineering
- Systems Analysis and Design
- Virtual Reality
- Browse content in Computing
- Business Applications
- Computer Security
- Computer Games
- Computer Networking and Communications
- Digital Lifestyle
- Graphical and Digital Media Applications
- Operating Systems
- Browse content in Earth Sciences and Geography
- Atmospheric Sciences
- Environmental Geography
- Geology and the Lithosphere
- Maps and Map-making
- Meteorology and Climatology
- Oceanography and Hydrology
- Palaeontology
- Physical Geography and Topography
- Regional Geography
- Soil Science
- Urban Geography
- Browse content in Engineering and Technology
- Agriculture and Farming
- Biological Engineering
- Civil Engineering, Surveying, and Building
- Electronics and Communications Engineering
- Energy Technology
- Engineering (General)
- Environmental Science, Engineering, and Technology
- History of Engineering and Technology
- Mechanical Engineering and Materials
- Technology of Industrial Chemistry
- Transport Technology and Trades
- Browse content in Environmental Science
- Applied Ecology (Environmental Science)
- Conservation of the Environment (Environmental Science)
- Environmental Sustainability
- Environmentalist Thought and Ideology (Environmental Science)
- Management of Land and Natural Resources (Environmental Science)
- Natural Disasters (Environmental Science)
- Nuclear Issues (Environmental Science)
- Pollution and Threats to the Environment (Environmental Science)
- Social Impact of Environmental Issues (Environmental Science)
- History of Science and Technology
- Browse content in Materials Science
- Ceramics and Glasses
- Composite Materials
- Metals, Alloying, and Corrosion
- Nanotechnology
- Browse content in Mathematics
- Applied Mathematics
- Biomathematics and Statistics
- History of Mathematics
- Mathematical Education
- Mathematical Finance
- Mathematical Analysis
- Numerical and Computational Mathematics
- Probability and Statistics
- Pure Mathematics
- Browse content in Neuroscience
- Cognition and Behavioural Neuroscience
- Development of the Nervous System
- Disorders of the Nervous System
- History of Neuroscience
- Invertebrate Neurobiology
- Molecular and Cellular Systems
- Neuroendocrinology and Autonomic Nervous System
- Neuroscientific Techniques
- Sensory and Motor Systems
- Browse content in Physics
- Astronomy and Astrophysics
- Atomic, Molecular, and Optical Physics
- Biological and Medical Physics
- Classical Mechanics
- Computational Physics
- Condensed Matter Physics
- Electromagnetism, Optics, and Acoustics
- History of Physics
- Mathematical and Statistical Physics
- Measurement Science
- Nuclear Physics
- Particles and Fields
- Plasma Physics
- Quantum Physics
- Relativity and Gravitation
- Semiconductor and Mesoscopic Physics
- Browse content in Psychology
- Affective Sciences
- Clinical Psychology
- Cognitive Psychology
- Cognitive Neuroscience
- Criminal and Forensic Psychology
- Developmental Psychology
- Educational Psychology
- Evolutionary Psychology
- Health Psychology
- History and Systems in Psychology
- Music Psychology
- Neuropsychology
- Organizational Psychology
- Psychological Assessment and Testing
- Psychology of Human-Technology Interaction
- Psychology Professional Development and Training
- Research Methods in Psychology
- Social Psychology
- Browse content in Social Sciences
- Browse content in Anthropology
- Anthropology of Religion
- Human Evolution
- Medical Anthropology
- Physical Anthropology
- Regional Anthropology
- Social and Cultural Anthropology
- Theory and Practice of Anthropology
- Browse content in Business and Management
- Business Ethics
- Business Strategy
- Business History
- Business and Technology
- Business and Government
- Business and the Environment
- Comparative Management
- Corporate Governance
- Corporate Social Responsibility
- Entrepreneurship
- Health Management
- Human Resource Management
- Industrial and Employment Relations
- Industry Studies
- Information and Communication Technologies
- International Business
- Knowledge Management
- Management and Management Techniques
- Operations Management
- Organizational Theory and Behaviour
- Pensions and Pension Management
- Public and Nonprofit Management
- Social Issues in Business and Management
- Strategic Management
- Supply Chain Management
- Browse content in Criminology and Criminal Justice
- Criminal Justice
- Criminology
- Forms of Crime
- International and Comparative Criminology
- Youth Violence and Juvenile Justice
- Development Studies
- Browse content in Economics
- Agricultural, Environmental, and Natural Resource Economics
- Asian Economics
- Behavioural Finance
- Behavioural Economics and Neuroeconomics
- Econometrics and Mathematical Economics
- Economic History
- Economic Systems
- Economic Methodology
- Economic Development and Growth
- Financial Markets
- Financial Institutions and Services
- General Economics and Teaching
- Health, Education, and Welfare
- History of Economic Thought
- International Economics
- Labour and Demographic Economics
- Law and Economics
- Macroeconomics and Monetary Economics
- Microeconomics
- Public Economics
- Urban, Rural, and Regional Economics
- Welfare Economics
- Browse content in Education
- Adult Education and Continuous Learning
- Care and Counselling of Students
- Early Childhood and Elementary Education
- Educational Equipment and Technology
- Educational Research Methodology
- Educational Strategies and Policy
- Higher and Further Education
- Organization and Management of Education
- Philosophy and Theory of Education
- Schools Studies
- Secondary Education
- Teaching of a Specific Subject
- Teaching of Specific Groups and Special Educational Needs
- Teaching Skills and Techniques
- Browse content in Environment
- Applied Ecology (Social Science)
- Climate Change
- Conservation of the Environment (Social Science)
- Environmentalist Thought and Ideology (Social Science)
- Management of Land and Natural Resources (Social Science)
- Natural Disasters (Environment)
- Pollution and Threats to the Environment (Social Science)
- Social Impact of Environmental Issues (Social Science)
- Sustainability
- Browse content in Human Geography
- Cultural Geography
- Economic Geography
- Political Geography
- Browse content in Interdisciplinary Studies
- Communication Studies
- Museums, Libraries, and Information Sciences
- Browse content in Politics
- African Politics
- Asian Politics
- Chinese Politics
- Comparative Politics
- Conflict Politics
- Elections and Electoral Studies
- Environmental Politics
- Ethnic Politics
- European Union
- Foreign Policy
- Gender and Politics
- Human Rights and Politics
- Indian Politics
- International Relations
- International Organization (Politics)
- International Political Economy
- Irish Politics
- Latin American Politics
- Middle Eastern Politics
- Political Behaviour
- Political Economy
- Political Institutions
- Political Methodology
- Political Communication
- Political Philosophy
- Political Sociology
- Political Theory
- Politics and Religion
- Politics and Law
- Politics of Development
- Public Policy
- Public Administration
- Qualitative Political Methodology
- Quantitative Political Methodology
- Regional Political Studies
- Russian Politics
- Security Studies
- State and Local Government
- UK Politics
- US Politics
- Browse content in Regional and Area Studies
- African Studies
- Asian Studies
- East Asian Studies
- Japanese Studies
- Latin American Studies
- Middle Eastern Studies
- Native American Studies
- Scottish Studies
- Browse content in Research and Information
- Research Methods
- Browse content in Social Work
- Addictions and Substance Misuse
- Adoption and Fostering
- Care of the Elderly
- Child and Adolescent Social Work
- Couple and Family Social Work
- Direct Practice and Clinical Social Work
- Emergency Services
- Human Behaviour and the Social Environment
- International and Global Issues in Social Work
- Mental and Behavioural Health
- Social Justice and Human Rights
- Social Policy and Advocacy
- Social Work and Crime and Justice
- Social Work Macro Practice
- Social Work Practice Settings
- Social Work Research and Evidence-based Practice
- Welfare and Benefit Systems
- Browse content in Sociology
- Childhood Studies
- Community Development
- Comparative and Historical Sociology
- Disability Studies
- Economic Sociology
- Gender and Sexuality
- Gerontology and Ageing
- Health, Illness, and Medicine
- Marriage and the Family
- Migration Studies
- Occupations, Professions, and Work
- Organizations
- Population and Demography
- Race and Ethnicity
- Social Theory
- Social Movements and Social Change
- Social Research and Statistics
- Social Stratification, Inequality, and Mobility
- Sociology of Religion
- Sociology of Education
- Sport and Leisure
- Urban and Rural Studies
- Browse content in Warfare and Defence
- Defence Strategy, Planning, and Research
- Land Forces and Warfare
- Military Administration
- Military Life and Institutions
- Naval Forces and Warfare
- Other Warfare and Defence Issues
- Peace Studies and Conflict Resolution
- Weapons and Equipment

- < Previous chapter
- Next chapter >

2 Visual Sensory Memory
- Published: August 2008
- Cite Icon Cite
- Permissions Icon Permissions
Visual stimuli not only remain visible for some time after their physical offset, but information about their characteristics also persists; that is, not only does something that looks like the physical stimulus continue to persist for a brief time after stimulus offset, but information can also be extracted from the stimulus for a brief time after its offset in much the same way as when the stimulus was physically present. This latter type of persistence is usually called informational persistence. Visible persistence and informational persistence reflect related but different aspects of visual sensory memory. This chapter discusses the evidence behind this assertion and describes the current conception of these phenomena.
Signed in as
Institutional accounts.
- GoogleCrawler [DO NOT DELETE]
- Google Scholar Indexing
Personal account
- Sign in with email/username & password
- Get email alerts
- Save searches
- Purchase content
- Activate your purchase/trial code
- Add your ORCID iD
Institutional access
Sign in with a library card.
- Sign in with username/password
- Recommend to your librarian
- Institutional account management
- Get help with access
Access to content on Oxford Academic is often provided through institutional subscriptions and purchases. If you are a member of an institution with an active account, you may be able to access content in one of the following ways:
IP based access
Typically, access is provided across an institutional network to a range of IP addresses. This authentication occurs automatically, and it is not possible to sign out of an IP authenticated account.
Choose this option to get remote access when outside your institution. Shibboleth/Open Athens technology is used to provide single sign-on between your institution’s website and Oxford Academic.
- Click Sign in through your institution.
- Select your institution from the list provided, which will take you to your institution's website to sign in.
- When on the institution site, please use the credentials provided by your institution. Do not use an Oxford Academic personal account.
- Following successful sign in, you will be returned to Oxford Academic.
If your institution is not listed or you cannot sign in to your institution’s website, please contact your librarian or administrator.
Enter your library card number to sign in. If you cannot sign in, please contact your librarian.
Society Members
Society member access to a journal is achieved in one of the following ways:
Sign in through society site
Many societies offer single sign-on between the society website and Oxford Academic. If you see ‘Sign in through society site’ in the sign in pane within a journal:
- Click Sign in through society site.
- When on the society site, please use the credentials provided by that society. Do not use an Oxford Academic personal account.
If you do not have a society account or have forgotten your username or password, please contact your society.
Sign in using a personal account
Some societies use Oxford Academic personal accounts to provide access to their members. See below.
A personal account can be used to get email alerts, save searches, purchase content, and activate subscriptions.
Some societies use Oxford Academic personal accounts to provide access to their members.
Viewing your signed in accounts
Click the account icon in the top right to:
- View your signed in personal account and access account management features.
- View the institutional accounts that are providing access.
Signed in but can't access content
Oxford Academic is home to a wide variety of products. The institutional subscription may not cover the content that you are trying to access. If you believe you should have access to that content, please contact your librarian.
For librarians and administrators, your personal account also provides access to institutional account management. Here you will find options to view and activate subscriptions, manage institutional settings and access options, access usage statistics, and more.
Our books are available by subscription or purchase to libraries and institutions.
- About Oxford Academic
- Publish journals with us
- University press partners
- What we publish
- New features
- Open access
- Rights and permissions
- Accessibility
- Advertising
- Media enquiries
- Oxford University Press
- Oxford Languages
- University of Oxford
Oxford University Press is a department of the University of Oxford. It furthers the University's objective of excellence in research, scholarship, and education by publishing worldwide
- Copyright © 2024 Oxford University Press
- Cookie settings
- Cookie policy
- Privacy policy
- Legal notice
This Feature Is Available To Subscribers Only
Sign In or Create an Account
This PDF is available to Subscribers Only
For full access to this pdf, sign in to an existing account, or purchase an annual subscription.
Academia.edu no longer supports Internet Explorer.
To browse Academia.edu and the wider internet faster and more securely, please take a few seconds to upgrade your browser .
Enter the email address you signed up with and we'll email you a reset link.
- We're Hiring!
- Help Center
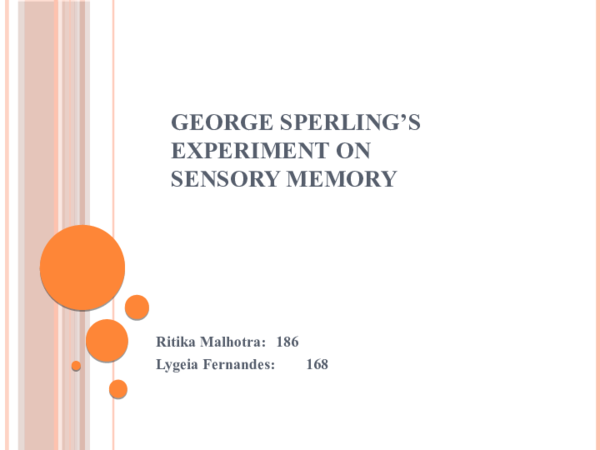
Download Free PDF
GEORGE SPERLING’S EXPERIMENT ON SENSORY MEMORY

Related papers
Handbook of Psychology, Second Edition, 2012
Memory is one of the oldest and most researched cognitive domains, and while much is established about verbal memory, research about visual memory is largely inconclusive. Past research acknowledges that visual memory is a complex function and research regarding visual perception, in particular the two streams hypothesis, clearly highlights an anatomical and functional demarcation between spatial and object processes. The current project consists of three experiments that investigate performance on a series of visual memory tasks that were designed to measure memory for spatial information and for object information separately. Each of the experiments extended upon the results and ideas that emerged from the previous experiment's findings.The purpose of the three experiments included: 1. The development and piloting of an electronic visual memory test battery that has clear assessment tasks that measure spatial and object abilities separately for short term, working and long ter...
This paper aims to introduce a new take on the concept of human memory. It is a preliminary take on this concept, and an experimental design on how the proposed hypothesis can be tested is also included. The paper starts out with a fresh overview of some essential neurological processes, and then introduces the fresh take. What follows is an experimental design based on the traditional Scientific Method, with each of its essential elements recognized promptly.
Neuropsychologia
Cognitive Psychology, 1973
Perception & Psychophysics, 1995
Memory & Cognition, 1977
Loading Preview
Sorry, preview is currently unavailable. You can download the paper by clicking the button above.
Historisk Tidsskrift, 1982
Revista Magister de Direito do Trabalho, 2022
2016. En: Juan Mauricio Renold (Comp.), Religión, Ciencias Sociales y Humanidades. Rosario: EditoraUNR, pp. .139-150
Canadian Military Journal , 2024
South African Journal of Physiotherapy, 1992
Health Psychology, 1988
PubMed, 2009
Social Anthropology, 2009
Kínesis, 2020
Westhoek, 2008
Journal of Thoracic Oncology, 2007
Journal of Periodontology, 2001
Summa Psicológica, 2013
- We're Hiring!
- Help Center
- Find new research papers in:
- Health Sciences
- Earth Sciences
- Cognitive Science
- Mathematics
- Computer Science
- Academia ©2024
404 Page Not Found

IMAGES
VIDEO
COMMENTS
The duration of sensory memory was first investigated during the 1960s by psychologist George Sperling. In a classic experiment, participants stared at a screen and rows of letters were flashed very briefly—for just 1/20th of a second.
George Sperling’s experiments provided crucial initial insight into the workings of sensory memory. Sensory memory is responsible for briefly storing information from our senses, such as sights, sounds, and smells, before being processed by our brains.
Eventually, in 1960, the American cognitive psychologist George Sperling, via several experiments, confirmed capacity and duration as parts of a memory system called visual sensory memory (Sperling, 1960).
Sperling documented the existence of iconic memory (one of the sensory memory subtypes). Through several experiments, he showed support for his hypothesis that human beings store a perfect image of the visual world for a brief moment, before it is discarded from memory.
Visual sensory memory, i.e., iconic memory, was first demonstrated by George Sperling using the partial-report paradigm (Sperling 1960): After a brief presentation of a 3 × 3 or 3 × 4 array of letters, observers often can report all the letters in any cued row if the cue occurs immediately after the visual presentation (“partial-report ...
By using the partial-report technique, Sperling showed that a large-capacity visual memory stores information for few hundred milliseconds (Sperling, 1960) and more recent studies indicate that this information is not implicit and unconscious but rather directly reflects the phenomenal richness of our visual experience (Vandenbroucke et al ...
In subsequent experiments, Sperling, Averbach and Coriell, and many other investigators discovered several important characteristics of this quickly decaying visual memory store. Several of these are described next.
The current project consists of three experiments that investigate performance on a series of visual memory tasks that were designed to measure memory for spatial information and for object information separately.
A model for visual recall tasks was presented in terms of visual information storage (VIS), scanning, rehearsal, and auditory information storage (AIS). It was shown first that brief visual stimuli are stored in VIS in a form similar to the sensory input.
You are now ready to investigate the sensory register (also known as iconic memory) by replicating several of Sperling’s (1960) experiments. Each experiment starts with whole report trials followed by trials in which report of a single row is cued.